11 Using Root-Soil Interactions in the Rhizosphere as Valuable Traits for Selection Against Drought
Abstract
The rhizosphere, meaning the soil volume influenced by the living roots, hosts several important ecological processes implicating the soil, the root system and active microbiota. These various interactions often impact soil carbon (C) content and nutrient dynamics, as well as soil water retention, by modifying its biological and physico-chemical properties. Well-known root adaptive traits for drought tolerance include deep rooting and increased root development, both of which ensure better exploration of the soil volume required for greater water uptake. However, the intensity of root-soil-microbiota interactions shape the size of the rhizosheath (i.e., the soil mass that remains attached to roots after plant excavation), which could modulate the water retention capacity of soil. Indeed, genotypes with larger rhizosheath respond better to drought stress than those with a smaller rhizosheath in several plant species. From a breeding perspective, intra-specific variation in rhizosheath size has recently been demonstrated in two important crops in West Africa: pearl millet and maize. Therefore, genotypes with large rhizosheath could be considered for varietal selection for adaptation to drought. Ongoing genome wide association studies (GWAS) should confirm genetic control of rhizosheath size and map candidate genes and investigations should be performed on the mechanisms that support this genetically complex trait.
Keywords: Rhizosphere, Rhizosheath, Root-adhering soil, Drought, Root phenotyping
Introduction
West Africa faces many challenges to secure sufficient and sustainable food production. Indeed, water scarcity and soil nutrient deficiency are among the main constraints limiting crop production. Drought stress is considered one of the most harmful abiotic stresses limiting crop yields (Fang et al., 2017; Praba et al., 2009; Wang et al., 2017). Moreover, drought events are projected to be exacerbated by climate change in much of the world including West-African countries (IPCC, 2018).
Plants explore the soil for water and for various nutrients they need for growth through their roots. They can then develop their root system architecture, associate with a beneficial microbiota, and improve the biological and physico-chemical conditions of the rhizosphere to face drought stress (White et al., 2013a, 2013b). Therefore, it may be possible to cope with drought by selecting crop varieties that can better exploit/use the soil water for sustainable production. The interactions between plant roots and soil that occur in the rhizosphere, defined as the volume of soil affected by the exudates secreted by plant roots and colonized by soil microbiota (Pinton et al., 2007), are very important to explore.
An important manifestation of the interactions between plant roots, soil, and root-associated microbiota, is the formation of the rhizosheath, or the aggregation of soil particles around the root after plant excavation (Brown et al., 2017; George et al., 2014; Pang et al., 2017). The rhizosheath is also the part of the rhizosphere where the root-soil-microbiota interactions are more intense and therefore it presents optimized physico-chemical conditions for water and mineral acquisition compared to bulk soil. Many factors contribute to its formation, including root physiology (e.g., exudation, association with soil microbiota), or morphology (e.g., root architecture, length, and density of root hair) (Ndour et al., 2020; Pang et al., 2017). Because of its role in plant growth under abiotic stress (including drought), this trait is now gaining attention of plant biologists, especially by those exploring the possibility of developing genetic tools for plant breeding, a now widely discussed topic regarding various crop species. Importantly, the formation of rhizosheath has been studied in pearl millet, the main cereal crop cultivated in West Africa (Ndour et al., 2017).
In this chapter, we will (1) discuss the relationship between plant rhizosphere functioning and drought stress; (2) analyse the intra-specific variability of the interactions in the rhizosphere for some important crops in West Africa and; (3) discuss the opportunity to integrate these root traits in plant breeding programs.
Response of the Rhizosphere to Drought Stress
Water is a crucial resource for plant development, and drought stress is the most damaging constraint for plant productivity (Leng & Hall, 2019). Through their roots, plants acquire water and minerals directly from the soil. The root system is of great importance in the interaction between crop and soil fertility as they are the main interface between the plant and the soil (Mommer et al., 2016). Moreover, water uptake depends on the soil’s physico-chemical characteristics, the physiology of plant roots and their morphological characteristics, as well as on the interactions between soil components and plant roots. Drought has several immediate consequences on plant physiology, including reduced seed germination rate, stomatal closure, reduced relative leaf water content and cell membrane stability, increased formation of reactive oxygen species, increased susceptibility to diseases, and reduced grain yield and quality (Zia et al., 2021). In the long term, plants develop various adaptation mechanisms to access water more efficiently. These include the development of several architectural and anatomical root traits such as root depth and aerenchyma formation (see Chapter 10, by Grondin et al. in this book), but also very interesting physiological traits which involve the soil matrix and its hosted microbiota (Grover et al., 2011; Lynch, 2011, 2019; Rodriguez et al., 2004).
The different interactions that take place in the plant-soil-microbe interface define rhizosphere functioning. These interactions are mediated by the root exudation (i.e., the deposition of organic compounds in the soil by plant roots). Root exudates are defined as the substances actively released by root in the soil from various mechanisms (Haichar et al., 2014; Nguyen, 2009; Sasse et al., 2018) and have a strong impact on the functioning of the rhizosphere (Cantó et al., 2020). Indeed, several studies have reported the influence of root exudation on the microbial composition of the rhizosphere in various crop species (Carvalhais et al., 2015; Ge et al., 2017; Haichar et al., 2008; Iannucci et al., 2021; Ma et al., 2018; Micallef et al., 2009; Neumann et al., 2014). Moreover, plant root exudation can be modulated in return by the rhizosphere microbiota (Canarini et al., 2019; Korenblum et al., 2020). Importantly, these interactions help plants to better withstand the various adverse effects of drought stress.
1. Plant Growth-promoting Microbiota
Microbial communities in the rhizosphere may be involved in plant response to drought. Under drought conditions, plant growth can be promoted by the direct response of soil microbiota as well as by indirect effects resulting from modification of root exudation (Vries et al., 2020). For instance, drought leads to a modification of the microbial community in the rhizosphere, including an increase in the relative abundance of Actinobacteria and Firmicutes, and a decrease of Proteobacteria and Bacteroidetes (Naylor & Coleman-Derr, 2018). In particular, a study on 30 species of angiosperms showed a significant correlation between the relative abundance of the root-colonizing Streptomyces and the drought tolerance of the host plant (Fitzpatrick et al., 2018). In this sense, a recent study on sorghum showed that drought stress increased the colonization of Streptomyces within the root and the rhizosphere, and that this colonization increases root development during drought (Xu et al., 2018). Recently, strains of Acinetobacter calcoaceticus and Penicillium sp isolated for their P-solubilization potential have been shown to mitigate the adverse effects of drought stress in foxtail millet (Kour et al., 2020). It will therefore be interesting to test on crop species cultivated in West Africa and their wild relatives, and to verify whether there is a genetic variation of rhizosphere and endosphere oligotrophic microbial communities that could help plants to better withstand drought conditions.
2. Arbuscular Mycorrhizal Fungi (AMF)
The association between plant roots and arbuscular mycorrhizal fungi is one of the most important plant symbiosis with soil microbiota (Cantó et al., 2020). It is widely recognised that plants associated with AMF have a greater water uptake capacity related to increased soil volume explored through the hyphal network. The positive effect of AMF on plant water uptake and plant drought tolerance was reported in both cereals and legumes. For example, inoculation of cowpea with the mycorrhizal fungus Glomus deserticola enabled the plant to withstand water stress and produced increased yield compared to the uninoculated control treatment (Oyewole et al., 2017). This positive effect was also observed in drought-stressed maize after inoculation with Glomus versiforme due to an improvement in the deleterious effects of drought-induced oxidative damage (Begum et al., 2019). In the case of pearl millet, mycorrhizal inoculation mitigated the effects of water deficit and helped the plants to maintain their biomass production (Fabbrin et al., 2015). So, these symbiotic associations are reliable opportunities and should be better explored for crop adaptation against drought in the West-African context.
3. Root Exudation and Rhizosheath Formation
Another striking and complex example of the physiological response of roots to drought is the formation of the rhizosheath around the root system. The rhizosheath is considered the most active compartment of the rhizosphere. The formation of the rhizosheath is an aggregation process responding to many factors including root exudation and production of bacterial exopolysaccharides (Ndour et al., 2020). Recently, it has been related to carbon exudation and dynamics in the rhizosphere at the early stages of development in pearl millet (Ndour et al., 2022). Price (1911) first hypothesized that plants developed rhizosheath formation as a mechanism to improve drought tolerance and root protection under arid conditions, a hypothesis that was recently confirmed (Alami et al., 2000; Benard et al., 2016; Pate & Dixon, 1996). Several other studies have reported a positive effect of a large rhizosheath in the growth of plants subjected to drought, such as foxtail millet and wheat (Basirat et al., 2019; T.-Y. Liu et al., 2018). A similar result was also obtained for a drought-tolerant chickpea in which Rabbi et al. (2018) showed greater water storage in the rhizosphere soil linked to a larger rhizosheath and greater exudation of root mucilage. Indeed, some of the factors affecting the rhizosheath, such as root exudation (mainly C exudation) and the associated exopolysaccharide production by rhizobacteria, increase organic matter content as well as soil aggregation in the rhizosphere (Alami et al., 2000; Berge et al., 2009). A recent study shows that moderate water stress leads to the formation of rhizsosheath in rice, and increased soil porosity and water content in the root-adhering soil volume compared to bulk soil (Zhang et al., 2020).
Overall, various studies have shown that the rhizosheath trait increased plant fitness, particularly under drought stress conditions. It can be assumed that the combination of better water retention due to the increase in organic matter content, particularly microbial polysaccharides, and improved soil structuration, which both occur in the rhizosheath, contributes to better water storage and availability in the immediate root periphery. Thus, genetic variability of these physiological traits on crop species could be very interesting from a plant breeding perspective to improve crop management, especially in arid regions. The various interactions taking place in the rhizosphere and contributing to drought stress tolerance are summarized in figure 1.
Figure 1
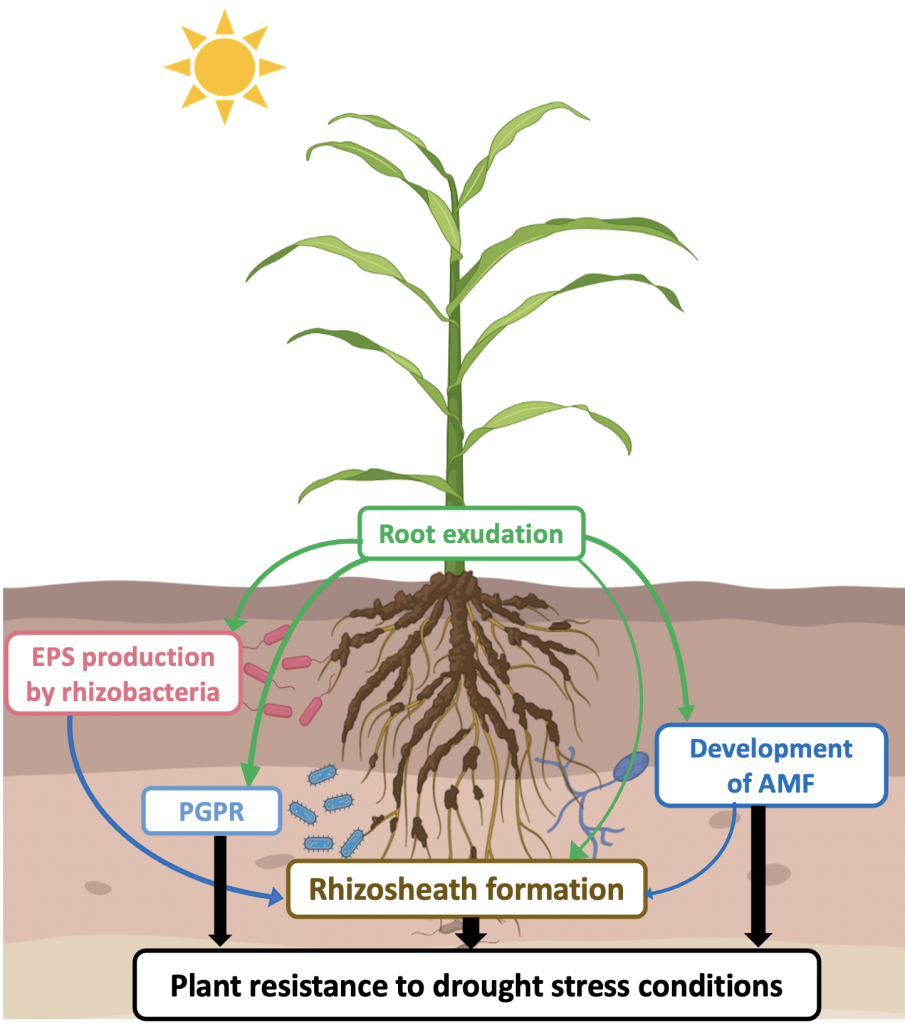
Note: EPS = Exopolysaccharide, PGPR = Plant Growth Promoting Rhizobacteria, AMF = Arbuscular Mycorrhizal Fungi
Genetic Variation of Rhizosphere Interactions in Crops: Example of the Rhizosheath
It is widely recognized that interactions in the rhizosphere play an important role in the response of plants to abiotic stresses and in particular in plant drought tolerance (Cantó et al. 2020). However, the relevant plant traits that characterize these interactions are often difficult to define due to their complexity. Consequently, intra-specific variations in these interactions are difficult to demonstrate. Nevertheless, the size of the rhizosheath has been used to test the genetic variability of the intensity of rhizosphere interactions in various species.
1. The Specific Rhizosheath Weight as a Proxy to Phenotype Rhizosphere Interactions
Different methods have been used to phenotype for rhizosheath size (reviewed in Ndour et al., 2020). The specific rhizosheath weight (i.e., the relative mass of dry soil that adheres firmly to the roots after plant has been excavated and roots have been shaken) is now widely considered as a reliable proxy to evaluate the rhizosheath size (Ndour et al., 2020). Operationally, it is defined as the ratio between the mass of dry root-adhering soil and the mass of dry root (RAS/RT). This emerging parameter was used to estimate the rhizosheath size on wheat (Amellal et al., 1998; Gouzou et al., 1993), sunflower (Alami et al., 2000; Sandhya et al., 2009), durum wheat (Kaci et al. 2005), barley (George et al. 2014) and on pearl millet (Ndour et al., 2017). Another parameter used to estimate the rhizosheath size is the ratio between the RAS dry mass and the total root length (RAS/RL), the latter of which was reported to be strongly correlated with the RAS/RT ratio (Adu et al., 2017). This method of estimating rhizosheath size by calculating the RAS/RT or the RAS/RL ratio seems to be effective for high-throughput phenotyping as it was used in numerous studies that aim to highlight its genetic determinants.
2. Case Study of the Phenotyping of Plant Rhizosphere Using the Rhizosheath Size
The rhizosheath size (RAS/RT or RAS/RL) would be genetically determined in many cereal species. First, phenotypic variation has been demonstrated in wheat (Delhaize et al., 2012; Haling et al., 2010; James et al., 2016), in barley (George et al., 2014; Gong & McDonald, 2017) and maize (Adu et al., 2017).
Our preliminary phenotyping study evaluated the impact of pearl millet genotypic variation on rhizosheath size using the RAS/RT ratio. Briefly, we grew 86 different inbred lines of pearl millet in MW pots filled with an arenosol sampled at the Centre National de Recherches Agronomiques (CNRA) of Bambey (Senegal), and each pot watered to its water holding capacity. After 28 days of growth, the plants were harvested and the root-adhering soil mass (RAS) and the root biomass (RT) were weighted and used to calculate the RAS/RT ratio, which was used as an estimator of rhizosheath size (Ndour et al., 2017). The results showed genotypic differences in pearl millet rhizosheath size. Subsequently, we replicated this experiment using the same protocol with a larger panel comprising 181 pearl millet lines for GWAS purposes. The results confirmed the genetic control of this trait within this larger panel of pearl millet lines with a 3-fold variation of the RAS/RT ratio between the more contrasting phenotypes (Ndour et al., 2021) and a broad heritability of 0.72 (Fig. 2).
Figure 2
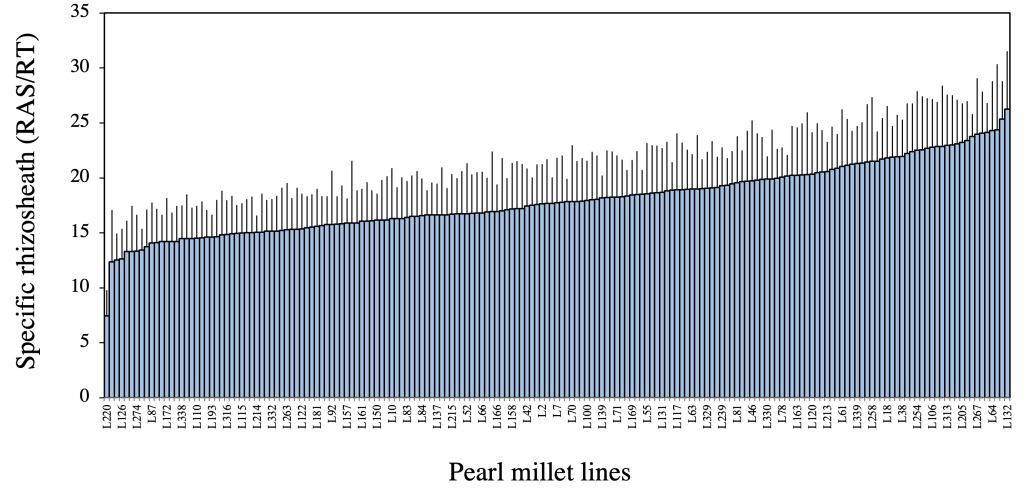
3. Microbial Diversity Phenotyping in the Rhizosphere of Pearl Millet
As mentioned above, this rhizosheath size variation can be explained by many factors. However, in a study we conducted in a set of 9 pearl millet inbred lines selected for their contrasting rhizosheath size (RAS/RT), we focused on the microbiota component and compared the diversity of bacterial communities in the rhizosphere. This study concluded that the size of this rhizosheath was related with bacterial diversity since, based on 16S rDNA sequences data, the relative abundances of some taxa including Proteobacteria and Firmicutes varied significantly between lines characterized by small and large rhizosheath (Fig. 3). Moreover, the -diversity indices (e.g., Shannon, Chao1, Richness) calculated from these sequences are higher for large rhizosheath lines compared to those with small rhizosheath. This finding confirmed the response of this trait to rhizosphere microbiota (Alami et al., 2000; Bezzate et al., 2000; Fernández Bidondo et al., 2012) and suggested its genetic control by the host plant, probably through root exudation, and its effect on root-soil-microbiota interaction.
Figure 3
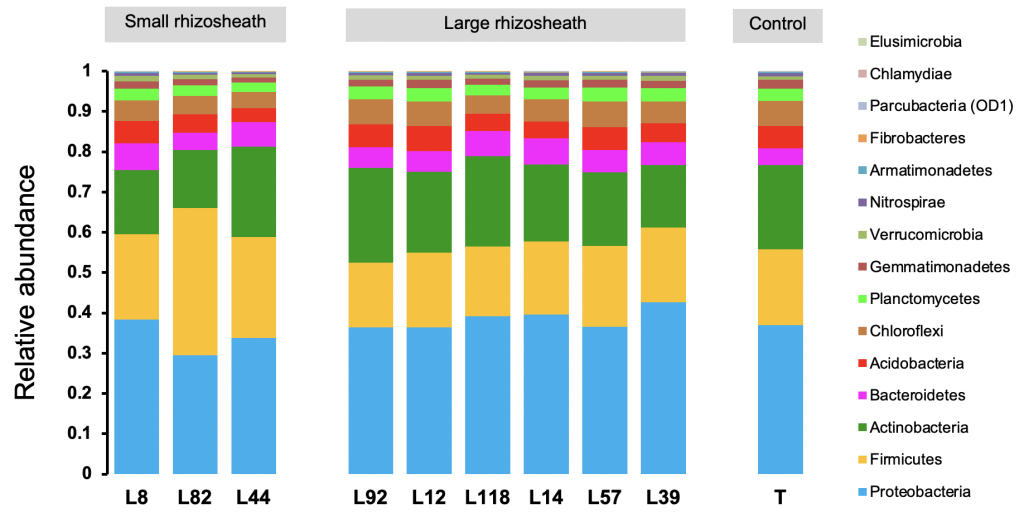
Another study we performed with contrasting rhizosheath pearl millet lines grown in the field showed that some enzymatic activities (e.g., chitinase, acid phosphomonoesterase, FDA hydrolysis) were higher in the root-adhering soil of the large rhizosheath lines compared to that of small rhizosheath lines (Ndour et al., 2021), suggesting that plant genetics (through root exudation variation) control not only the structure of the microbial community but also their enzymatic activities. This finding confirms the importance of root-soil-microbiota interactions, notably the importance of rhizosheath formation in C, nutrient dynamics in the rhizosphere, and consequently in plant nutrition under abiotic stress such as drought.
Opportunities for Plant Breeding for Drought Stress Tolerance
The potential of targeting root functioning for plant improvement against abiotic stress is well documented (Cantó et al., 2020; Lynch, 2019). This is particularly relevant in arid and semi-arid regions of West Africa where plants face multiple constraints including drought stress (Dossa et al., 2017; Drabo et al., 2019). Genetic variations in these root traits could be studied for the major crops of the region in order to ensure sustainable crop production.
For the rhizosheath trait for which genetic variations have been found in various crops, QTLs have been already detected in wheat (Delhaize et al., 2012; James et al., 2016; M. Liu et al., 2017) and barley (George et al., 2014; Gong & McDonald, 2017), although the molecular mechanisms explaining these phenotypes remain unknown. Adu et al. (2017) showed that rhizosheath was larger in maize improved varieties compared to landraces; based on these results, one may hypothesize that GWAS with a larger collection could therefore be used to detect QTLs and controlling genes in this important cereal for West-African countries. This trait should be further investigated for the other common cereals in West Africa such as sorghum. For pearl millet, the QTLs controlling this rhizosheath trait remain to be detected. However, the availibity of a reference pearl millet genome which was recently sequenced (Varshney et al., 2017) should make possible the rapid determination of the candidate genes related to this trait.
Conclusion
Drought is the main abiotic stress negatively affecting crop yield via plant growth, health, and reproduction (Fahad et al., 2017; Lamaoui et al., 2018; Leng & Hall, 2019). In response to this constraint, plants adapt their root morphology but also develop complex interactions with the soil and the root-associated microbiota. In the case of root interactions, the formation of rhizosheath improves plant drought tolerance through the combination of (1) exploration of a larger soil volume and (2) better water retention capacity due to increased organic matter content. The genetic variability of these root traits observed in pearl millet opens new opportunities for genetic selection to support crop and food production resilience in West Africa.
Acknowledgements
This work was supported by the NewPearl grant (co-funded by Agropolis Fondation [project N° AF 1301-015, as part of the “Programme Investissement d’Avenir” ANR-l0-LABX-0001-0 l] and Fondazione Cariplo [N° FC 2013-0891]). We acknowledge the CORAF through the West Africa Agricultural Productivity Programme (WAAPP) and the Ministry of higher education and research of Senegal government for the financial support allocated to PMSN during his doctoral studies. We also thank the French National Research Institute for Sustainable Development (IRD) and the Make Our Planet Great Again (MOPGA) initiative of the French government for co-funding the postdoctoral fellowship of PMSN.
References
Adu, M. O., Asare, P. A., Yawson, D. O., Ackah, F. K., Amoah, K. K., Nyarko, M. A., & Andoh, D. A. (2017). Quantifying variations in rhizosheath and root system phenotypes of landraces and improved varieties of juvenile maize. Rhizosphere, 3(1), 29–39. https://doi.org/10.1016/j.rhisph.2016.12.004
Alami, Y., Achouak, W., Marol, C., & Heulin, T. (2000). Rhizosphere soil aggregation and plant growth promotion of sunflowers by an exopolysaccharide-producing Rhizobiumsp. Strain isolated from sunflower roots. Applied and Environmental Microbiology, 66(8), 3393–3398. https://doi.org/10.1128/AEM.66.8.3393-3398.2000
Amellal, N., Burtin, G., Bartoli, F., & Heulin, T. (1998). Colonization of wheat roots by an exopolysaccharide-producing Pantoea agglomerans strain and its effect on rhizosphere soil aggregation. Applied and Environmental Microbiology, 64(10), 3740–3747.
Basirat, M., Mousavi, S. M., Abbaszadeh, S., Ebrahimi, M., & Zarebanadkouki, M. (2019). The rhizosheath: A potential root trait helping plants to tolerate drought stress. Plant and Soil, 445, 565–575. https://doi.org/10.1007/s11104-019-04334-0
Begum, N., Ahanger, M. A., Su, Y., Lei, Y., Mustafa, N. S. A., Ahmad, P., & Zhang, L. (2019). Improved drought tolerance by AMF inoculation in maize (Zea mays) involves physiological and biochemical implications. Plants, 8(12), 579. https://doi.org/10.3390/plants8120579
Benard, P., Kroener, E., Vontobel, P., Kaestner, A., & Carminati, A. (2016). Water percolation through the root-soil interface. Advances in Water Resources, 95, 190–198. https://doi.org/10.1016/j.advwatres.2015.09.014
Berge, O., Lodhi, A., Brandelet, G., Santaella, C., Roncato, M.-A., Christen, R., Heulin, T., & Achouak, W. (2009). Rhizobium alamii sp. Nov., an exopolysaccharide-producing species isolated from legume and non-legume rhizospheres. International Journal of Systematic and Evolutionary Microbiology, 59(2), 367–372. https://doi.org/10.1099/ijs.0.000521-0
Bezzate, S., Aymerich, S., Chambert, R., Czarnes, S., Berge, O., & Heulin, T. (2000). Disruption of the Paenibacillus polymyxa levansucrase gene impairs its ability to aggregate soil in the wheat rhizosphere. Environmental Microbiology, 2(3), 333–342. https://doi.org/10.1046/j.1462-2920.2000.00114.x
Brown, L. K., George, T. S., Neugebauer, K., & White, P. J. (2017). The rhizosheath – a potential trait for future agricultural sustainability occurs in orders throughout the angiosperms. Plant and Soil, 418(1), 115–128. https://doi.org/10.1007/s11104-017-3220-2
Canarini, A., Kaiser, C., Merchant, A., Richter, A., & Wanek, W. (2019). Root exudation of primary metabolites: Mechanisms and their roles in plant responses to environmental stimuli. Frontiers in Plant Science, 10. https://doi.org/10.3389/fpls.2019.00157
Cantó, C. de la F., Simonin, M., King, E., Moulin, L., Bennett, M. J., Castrillo, G., & Laplaze, L. (2020). An extended root phenotype : The rhizosphere, its formation and impacts on plant fitness. The Plant Journal, 103(3), 951–964. https://doi.org/10.1111/tpj.14781
Carvalhais, L. C., Dennis, P. G., Badri, D. V., Kidd, B. N., Vivanco, J. M., & Schenk, P. M. (2015). Linking jasmonic acid signaling, root exudates, and rhizosphere microbiomes. Molecular Plant-Microbe Interactions, 28(9), 1049–1058. https://doi.org/10.1094/MPMI-01-15-0016-R
Delhaize, E., James, R. A., & Ryan, P. R. (2012). Aluminium tolerance of root hairs underlies genotypic differences in rhizosheath size of wheat (Triticum aestivum) grown on acid soil. New Phytologist, 195(3), 609–619. https://doi.org/10.1111/j.1469-8137.2012.04183.x
Dossa, K., Konteye, M., Niang, M., Doumbia, Y., & Cissé, N. (2017). Enhancing sesame production in West Africa’s Sahel: A comprehensive insight into the cultivation of this untapped crop in Senegal and Mali. Agriculture & Food Security, 6(1). https://doi.org/10.1186/s40066-017-0143-3
Drabo, I., Zangre, R. G., Danquah, E. Y., Ofori, K., Witcombe, J. R., & Hash, C. T. (2019). Identifying farmers’ preferences and constraints to pearl millet production in the Sahel and north-Sudan zones of Burkina Faso. Experimental Agriculture, 55(5), 765–775. https://doi.org/10.1017/S0014479718000352
Fabbrin, E. G., Gogorcena, Y., Mogor, Á. F., Garmendia, I., & Goicoechea, N. (2015). Pearl millet growth and biochemical alterations determined by mycorrhizal inoculation, water availability and atmospheric CO2 concentration. Crop and Pasture Science, 66(8), 831–840. https://doi.org/10.1071/CP14089
Fahad, S., Bajwa, A. A., Nazir, U., Anjum, S. A., Farooq, A., Zohaib, A., Sadia, S., Nasim, W., Adkins, S., Saud, S., Ihsan, M. Z., Alharby, H., Wu, C., Wang, D., & Huang, J. (2017). Crop production under drought and heat stress: Plant responses and management options. Frontiers in Plant Science, 8, 1147. https://doi.org/10.3389/fpls.2017.01147
Fang, Y., Du, Y., Wang, J., Wu, A., Qiao, S., Xu, B., Zhang, S., Siddique, K. H. M., & Chen, Y. (2017). Moderate drought stress affected root growth and grain yield in old, modern and newly released cultivars of winter wheat. Frontiers in Plant Science, 8, 672. https://doi.org/10.3389/fpls.2017.00672
Fernández Bidondo, L., Bompadre, J., Pergola, M., Silvani, V., Colombo, R., Bracamonte, F., & Godeas, A. (2012). Differential interaction between two Glomus intraradices strains and a phosphate solubilizing bacterium in maize rhizosphere. Pedobiologia, 55(4), 227–232. https://doi.org/10.1016/j.pedobi.2012.04.001
Fitzpatrick, C. R., Copeland, J., Wang, P. W., Guttman, D. S., Kotanen, P. M., & Johnson, M. T. J. (2018). Assembly and ecological function of the root microbiome across angiosperm plant species. Proceedings of the National Academy of Sciences, 115(6), E1157–E1165. https://doi.org/10.1073/pnas.1717617115
Ge, T., Wei, X., Razavi, B. S., Zhu, Z., Hu, Y., Kuzyakov, Y., Jones, D. L., & Wu, J. (2017). Stability and dynamics of enzyme activity patterns in the rice rhizosphere: Effects of plant growth and temperature. Soil Biology and Biochemistry, 113, 108–115. https://doi.org/10.1016/j.soilbio.2017.06.005
George, T. S., Brown, L. K., Ramsay, L., White, P. J., Newton, A. C., Bengough, A. G., Russell, J., & Thomas, W. T. B. (2014). Understanding the genetic control and physiological traits associated with rhizosheath production by barley (Hordeum vulgare). New Phytologist, 203(1), 195–205. https://doi.org/10.1111/nph.12786
Gong, X., & McDonald, G. (2017). QTL mapping of root traits in phosphorus-deficient soils reveals important genomic regions for improving NDVI and grain yield in barley. Theoretical and Applied Genetics, 130(9), 1885–1902. https://doi.org/10.1007/s00122-017-2931-3
Gouzou, L., Burtin, G., Philippy, R., Bartoli, F., & Heulin, T. (1993). Effect of inoculation with Bacillus polymyxa on soil aggregation in the wheat rhizosphere: Preliminary examination. Geoderma, 56(1–4), 479–491. https://doi.org/10.1016/0016-7061(93)90128-8
Grover, M., Ali, S. Z., Sandhya, V., Rasul, A., & Venkateswarlu, B. (2011). Role of microorganisms in adaptation of agriculture crops to abiotic stresses. World Journal of Microbiology and Biotechnology, 27(5), 1231–1240. https://doi.org/10.1007/s11274-010-0572-7
Haichar, F. el Z., Marol, C., Berge, O., Rangel-Castro, J. I., Prosser, J. I., Balesdent, J., Heulin, T., & Achouak, W. (2008). Plant host habitat and root exudates shape soil bacterial community structure. The ISME Journal, 2(12), 1221–1230. https://doi.org/10.1038/ismej.2008.80
Haichar, F. el Z., Santaella, C., Heulin, T., & Achouak, W. (2014). Root exudates mediated interactions belowground. Soil Biology and Biochemistry, 77(Supplement C), 69–80. https://doi.org/10.1016/j.soilbio.2014.06.017
Haling, R. E., Simpson, R. J., Delhaize, E., Hocking, P. J., & Richardson, A. E. (2010). Effect of lime on root growth, morphology and the rhizosheath of cereal seedlings growing in an acid soil. Plant and Soil, 327(1‑2), 199–212. https://doi.org/10.1007/s11104-009-0047-5
Iannucci, A., Canfora, L., Nigro, F., De Vita, P., & Beleggia, R. (2021). Relationships between root morphology, root exudate compounds and rhizosphere microbial community in durum wheat. Applied Soil Ecology, 158, 103781. https://doi.org/10.1016/j.apsoil.2020.103781
IPCC. (2018). Global warming Global warming of 1.5°C In : V Masson‐Delmotte, P Zhai, HO Pörtner, D Roberts, J Skea, PR Shukla, A Pirani, W Moufouma‐Okia, C Péan, R Pidcock, S Connors, JBR Matthews, Y Chen, X Zhou, MI Gomis, E Lonnoy, T Maycock, M Tignor, T Waterfield, eds. An IPCC Special Report on the impacts of global warming of 1.5°C above pre‐industrial levels and related global greenhouse gas emission pathways, in the context of strengthening the global response to the threat of climate change, sustainable development, and efforts to eradicate poverty. [Technical report]. Cambridge University Press. https://www.ipcc.ch/site/assets/uploads/sites/2/2018/12/SR15_TS_High_Res.pdf
James, R. A., Weligama, C., Verbyla, K., Ryan, P. R., Rebetzke, G. J., Rattey, A., Richardson, A. E., & Delhaize, E. (2016). Rhizosheaths on wheat grown in acid soils: Phosphorus acquisition efficiency and genetic control. Journal of Experimental Botany, 67(12), 3709–3718. https://doi.org/10.1093/jxb/erw035
Kaci, Y., Heyraud, A., Barakat, M., & Heulin, T. (2005). Isolation and identification of an EPS-producing Rhizobium strain from arid soil (Algeria) : Characterization of its EPS and the effect of inoculation on wheat rhizosphere soil structure. Research in Microbiology, 156(4), 522–531. https://doi.org/10.1016/j.resmic.2005.01.012
Korenblum, E., Dong, Y., Szymanski, J., Panda, S., Jozwiak, A., Massalha, H., Meir, S., Rogachev, I., & Aharoni, A. (2020). Rhizosphere microbiome mediates systemic root metabolite exudation by root-to-root signaling. Proceedings of the National Academy of Sciences, 117(7), 3874–3883. https://doi.org/10.1073/pnas.1912130117
Kour, D., Rana, K. L., Yadav, A. N., Sheikh, I., Kumar, V., Dhaliwal, H. S., & Saxena, A. K. (2020). Amelioration of drought stress in Foxtail millet (Setaria italica L.) by P-solubilizing drought-tolerant microbes with multifarious plant growth promoting attributes. Environmental Sustainability, 3(1), 23–34. https://doi.org/10.1007/s42398-020-00094-1
Lamaoui, M., Jemo, M., Datla, R., & Bekkaoui, F. (2018). Heat and drought stresses in crops and approaches for their mitigation. Frontiers in Chemistry, 6, 26. https://doi.org/10.3389/fchem.2018.00026
Leng, G., & Hall, J. (2019). Crop yield sensitivity of global major agricultural countries to droughts and the projected changes in the future. Science of the Total Environment, 654, 811–821. https://doi.org/10.1016/j.scitotenv.2018.10.434
Liu, M., Rathjen, T., Weligama, K., Forrest, K., Hayden, M., & Delhaize, E. (2017). Analysis of aneuploid lines of bread wheat to map chromosomal locations of genes controlling root hair length. Annals of Botany, 119(8), 1333–1341. https://doi.org/10.1093/aob/mcx030
Liu, T.-Y., Ye, N., Song, T., Cao, Y., Gao, B., Zhang, D., Zhu, F., Chen, M., Zhang, Y., Xu, W., & Zhang, J. (2018). Rhizosheath formation and involvement in foxtail millet (Setaria italica) root growth under drought stress. Journal of Integrative Plant Biology, 61(4), 449–462. https://doi.org/10.1111/jipb.12716
Lynch, J. P. (2011). Root phenes for enhanced soil exploration and phosphorus acquisition: Tools for future crops. Plant Physiology, 156(3), 1041–1049. https://doi.org/10.1104/pp.111.175414
Lynch, J. P. (2019). Root phenotypes for improved nutrient capture: An underexploited opportunity for global agriculture. New Phytologist, 223(2), 548–564. https://doi.org/10.1111/nph.15738
Ma, X., Zarebanadkouki, M., Kuzyakov, Y., Blagodatskaya, E., Pausch, J., & Razavi, B. S. (2018). Spatial patterns of enzyme activities in the rhizosphere : Effects of root hairs and root radius. Soil Biology and Biochemistry, 118, 69–78. https://doi.org/10.1016/j.soilbio.2017.12.009
Micallef, S. A., Shiaris, M. P., & Colón-Carmona, A. (2009). Influence of Arabidopsis thaliana accessions on rhizobacterial communities and natural variation in root exudates. Journal of Experimental Botany, 60(6), 1729–1742. https://doi.org/10.1093/jxb/erp053
Mommer, L., Hinsinger, P., Prigent-Combaret, C., & Visser, E. J. W. (2016). Advances in the rhizosphere: Stretching the interface of life. Plant and Soil, 407(1/2), 1–8. https://doi.org/10.1007/s11104-016-3040-9
Naylor, D., & Coleman-Derr, D. (2018). Drought stress and root-associated bacterial communities. Frontiers in Plant Science, 8, 2223. https://doi.org/10.3389/fpls.2017.02223
Ndour, P. M. S., Barry, C. M., Tine, D., De la Fuente Cantó, C., Gueye, M., Barakat, M., Ortet, P., Achouak, W., Ndoye, I., Sine, B., Laplaze, L., Heulin, T., & Cournac, L. (2021). Pearl millet genotype impacts microbial diversity and enzymatic activities in relation to root-adhering soil aggregation. Plant and Soil. https://doi.org/10.1007/s11104-021-04917-w
Ndour, P. M. S., Gueye, M., Barakat, M., Ortet, P., Bertrand-Huleux, M., Pablo, A.-L., Dezette, D., Chapuis-Lardy, L., Assigbetsé, K., Kane, N., Yves, V., Achouak, W., Ndoye, I., Heulin, T., & Cournac, L. (2017). Pearl millet genetic traits shape rhizobacterial diversity and modulate rhizosphere aggregation. Frontiers in Plant Science, 8, 1288. https://doi.org/10.3389/fpls.2017.01288
Ndour, P. M. S., Hatté, C., Achouak, W., Heulin, T., & Cournac, L. (2022). Rhizodeposition efficiency of pearl millet genotypes assessed on short growing period by carbon isotopes (δ13C and F14C). Soil, 8(1), 49–57. https://doi.org/10.5194/soil-8-49-2022
Ndour, P. M. S., Heulin, T., Achouak, W., Laplaze, L., & Cournac, L. (2020). The rhizosheath: From desert plants adaptation to crop breeding. Plant and Soil, 456(1–2), 1–13. https://doi.org/10.1007/s11104-020-04700-3
Neumann, G., Bott, S., Ohler, M. A., Mock, H.-P., Lippmann, R., Grosch, R., & Smalla, K. (2014). Root exudation and root development of lettuce (Lactuca sativa L. cv. Tizian) as affected by different soils. Frontiers in Microbiology, 5, 2. https://doi.org/10.3389/fmicb.2014.00002
Nguyen, C. (2009). Rhizodeposition of organic C by plant: Mechanisms and controls. In Sustainable Agriculture (p. 97–123). Springer. https://link.springer.com/chapter/10.1007/978-90-481-2666-8_9
Oyewole, B. O., Olawuyi, O. J., Odebode, A. C., & Abiala, M. A. (2017). Influence of Arbuscular mycorrhiza fungi (AMF) on drought tolerance and charcoal rot disease of cowpea. Biotechnology Reports, 14, 8–15. https://doi.org/10.1016/j.btre.2017.02.004
Pang, J., Ryan, M. H., Siddique, K. H. M., & Simpson, R. J. (2017). Unwrapping the rhizosheath. Plant and Soil, 418(1‑2), 129–139. https://doi.org/10.1007/s11104-017-3358-y
Pate, J., & Dixon, K. W. (1996). Convergence and divergence in the southwestern Australian flora in adaptations of roots to limited availability of water and nutrients, fire and heat stress. In S. D. Hopper, J. A. Chappill, M. S. Harvey, & A. S. George (Eds.), Gondwanan Heritage (pp. 249–258). Surrey Beatty & Sons.
Pinton, R., Varanini, Z., & Nannipieri, P. (Eds.). (2007). The rhizosphere: Biochemistry and organic substances at the soil-plant interface (2nd ed.). CRC Press.
Praba, M. L., Cairns, J. E., Babu, R. C., & Lafitte, H. R. (2009). Identification of physiological traits underlying cultivar differences in drought tolerance in rice and wheat. Journal of Agronomy and Crop Science, 195(1), 30–46. https://doi.org/10.1111/j.1439-037X.2008.00341.x
Price, S. R. (1911). The roots of some north African desert-grasses. New Phytologist, 10(9‑10), 328–340. https://doi.org/10.1111/j.1469-8137.1911.tb06524.x
Rabbi, S. M. F., Tighe, M. K., Flavel, R. J., Kaiser, B. N., Guppy, C. N., Zhang, X., & Young, I. M. (2018). Plant roots redesign the rhizosphere to alter the three-dimensional physical architecture and water dynamics. New Phytologist, 219(2), 542–550. https://doi.org/10.1111/nph.15213
Rodriguez, R. J., Redman, R. S., & Henson, J. M. (2004). The role of fungal symbioses in the adaptation of plants to high stress environments. Mitigation and Adaptation Strategies for Global Change, 9(3), 261–272. https://doi.org/10.1023/B:MITI.0000029922.31110.97
Sandhya, V., SK. Z, A., Grover, M., Reddy, G., & Venkateswarlu, B. (2009). Alleviation of drought stress effects in sunflower seedlings by the exopolysaccharides producing Pseudomonas putida strain GAP-P45. Biology and Fertility of Soils, 46(1), 17–26. https://doi.org/10.1007/s00374-009-0401-z
Sasse, J., Martinoia, E., & Northen, T. (2018). Feed your friends: Do plant exudates shape the root microbiome? Trends in Plant Science, 23(1), 25–41. https://doi.org/10.1016/j.tplants.2017.09.003
Varshney, R. K., Shi, C., Thudi, M., Mariac, C., Wallace, J., Qi, P., Zhang, H., Zhao, Y., Wang, X., Rathore, A., Srivastava, R. K., Chitikineni, A., Fan, G., Bajaj, P., Punnuri, S., Gupta, S. K., Wang, H., Jiang, Y., Couderc, M., … Xu, X. (2017). Pearl millet genome sequence provides a resource to improve agronomic traits in arid environments. Nature Biotechnology, 36 (4), 368. https://doi.org/10.1038/nbt.3943
Vries, F. T. de, Griffiths, R. I., Knight, C. G., Nicolitch, O., & Williams, A. (2020). Harnessing rhizosphere microbiomes for drought-resilient crop production. Science, 368(6488), 270–274. https://doi.org/10.1126/science.aaz5192
Wang, J.-Y., Xiong, Y.-C., Li, F.-M., Siddique, K. H. M., & Turner, N. C. (2017). Effects of drought stress on morphophysiological traits, biochemical characteristics, yield, and yield components in different ploidy wheat: A meta-analysis. In D. L. Sparks (Éd.), Advances in agronomy (Vol. 143, pp. 139–173). Academic Press.
White, P. J., George, T. S., Dupuy, L. X., Karley, A. J., Valentine, T. A., Wiesel, L., & Wishart, J. (2013a). Root traits for infertile soils. Frontiers in Plant Science, 4, 193. https://doi.org/10.3389/fpls.2013.00193
White, P. J., George, T. S., Gregory, P. J., Bengough, A. G., Hallett, P. D., & McKenzie, B. M. (2013b). Matching roots to their environment. Annals of Botany, 112(2), 207–222. https://doi.org/10.1093/aob/mct123
Xu, L., Naylor, D., Dong, Z., Simmons, T., Pierroz, G., Hixson, K. K., Kim, Y.-M., Zink, E. M., Engbrecht, K. M., Wang, Y., Gao, C., DeGraaf, S., Madera, M. A., Sievert, J. A., Hollingsworth, J., Birdseye, D., Scheller, H. V., Hutmacher, R., Dahlberg, J., … Coleman-Derr, D. (2018). Drought delays development of the sorghum root microbiome and enriches for monoderm bacteria. Proceedings of the National Academy of Sciences, 115(18), E4284–E4293. https://doi.org/10.1073/pnas.1717308115
Zhang, Y., Du, H., Gui, Y., Xu, F., Liu, J., Zhang, J., & Xu, W. (2020). Moderate water stress in rice induces rhizosheath formation associated with abscisic acid and auxin responses. Journal of Experimental Botany, 71(9), 2740–2751. https://doi.org/10.1093/jxb/eraa021
Zia, R., Nawaz, M. S., Siddique, M. J., Hakim, S., & Imran, A. (2021). Plant survival under drought stress: Implications, adaptive responses, and integrated rhizosphere management strategy for stress mitigation. Microbiological Research, 242, 126626. https://doi.org/10.1016/j.micres.2020.126626