18 Genetic Improvement of Pearl Millet in Senegal: Past, Present and Future Prospects
Abstract
Pearl millet is an important cereal crop for smallholder farmers’ food security in Senegal and is grown on approximately 800,000 ha of land with a total national production of 600,000 tons annually. However, its production has been affected by increasing biotic and abiotic stresses such as downy mildew disease, drought and heat, and use of unimproved varieties in farmers’ fields. Genetic improvement of pearl millet started in Senegal nearly a century ago and experienced three major phases that were defined by different improvement targets. Over time, Progressive national yield improvements have been made. This chapter summarizes the breeding progress of the Senegal national breeding program in the past century. It also formulates future directions for further improvement of the productivity of pearl millet to meet the increasing national demand for food and nutrition security in the midst of climate change. This chapter posits that an ambitious pearl millet improvement strategy that integrates the modern genetic and genomic tools and novel genetic resources for resistance to biotic and abiotic stresses with variety development work is urgently needed to revolutionize pearl millet production in Senegal.
Keywords: pearl millet, drought adaptation, genetic improvement, Senegal
Introduction
Pearl millet [Pennisetum glaucum (L.) R. Br. syn. Cenchrus americanus (L.)] is a diploid (2x = 2n = 14) C4 and allogamous crop that belongs to the family of Poaceae (Bono & Leclercq, 1963). Archaeological and genetic findings have inferred PM was domesticated about 4,900 years ago in the western Sahara belt, more precisely in Northern Mali (Burgarella et al., 2018; Manning et al., 2011; Oumar et al., 2008).
Pearl millet is currently the sixth most important cereal crop in the world following maize (Zea mays), rice (Oryza sativa), wheat (Triticum aestivum), barley (Hordium vulgare), and sorghum (Sorghum bicolor) based on harvested area (FAOSTAT, 2019). It accounts for more than half of global millet production and is mainly cultivated in West Africa and India for household food security and income generation, whereas it is cultivated mostly as forage in Brazil, the United States, Mexico, and Australia. West Africa and India cover more than 95% of its global production (FAOSTAT, 2019). India is the largest pearl millet producer, followed by West African countries including Nigeria, Niger, Burkina Faso, Chad, Mali, and Senegal.
In Sub-Saharan Africa, pearl millet was historically grown by farmers for their basic subsistence. Now, it has also become a commercial crop and the main source of income and nutrients in the most drought and heat-stressed production areas. It is one of the rare cereals of traditional farming systems that is able to withstand frequent and prolonged periods of drought and high temperature in saline soils, at both the vegetative and reproductive phases, which would cause crop failure or greater yield reduction in other cereals (Dussert et al., 2015; Serba et al., 2017). Compared to some other domesticated cereals, pearl millet shows a large genetic diversity and an exceptional potential of adaptation to a wide range of ecological conditions, especially drought, across the semiarid areas (Sehgal et al., 2015; Serba et al. 2019).
Another major asset of pearl millet is its potential to relieve malnutrition in developing countries. Food insecurity remains the major issue to be solved in Sub-Saharan Africa, where 22% of the population is severely food insecure (ANSD, 2018). Despite improvements over the last decade, the current food insecurity situation in Senegal remains critical, and 16.5% of the population was undernourished in 2018 (ANSD, 2018). The food demand will increase dramatically in the coming years following continued population growth, with the projected West African population (920 million in mid-2014) doubling by 2050. The Senegalese population is expected to reach 40 million by 2050 (FAOSTAT, 2019). Pearl millet significantly helps mitigate malnutrition, as it is more nutritious than maize, rice, wheat, and sorghum because its grain contains higher levels of protein, vitamins, amino acids, antioxidants, fibers, and essential micronutrients (Saleh et al., 2013; Serba et al., 2017). Additionally, this crop has a high potential for transformation into value-added products. Pearl millet grain is used to prepare many traditional foods and is consumed in both rural and urban areas in many forms, such as porridges, gruels, doughs, couscous, and breads. It is also used for forage purposes.
All actors in the Senegalese pearl millet value chain recognized the need to develop strategies to substantially enhance grain yield. Therefore, the breeding program is under time pressure to act as a bridge between the market demand and the crop productivity and should rapidly adopt an efficient breeding strategy to accelerate the improvement of new varieties with better resilience to climate variability in particular drought stress. This chapter highlights the progress in pearl millet breeding in the last several decades, identifies research gaps, and formulates actionable future strategies for improvement of this nationally important cereal crop, particularly in the context of climate change.
Status and Constraints for Pearl Millet Cultivation in Senegal
Pearl millet is the main staple cereal crop in Senegal, with approximately 800,000 ha of growing area, occupying about 60% of the crop area harvested (FAOSTAT, 2019). It is mainly cultivated in the central, southern, and south-eastern parts of the country, more specifically in the Fatick, Kaffrine, Kaolack, Diourbel, Thies, Tambacounda, and Kolda regions (Figure 1). In 2019, its production in these regions represented more than 80% of total national production (ANSD, 2020). As the populations living in these regions depend particularly on pearl millet production for their livelihoods, improved production technologies are highly sought.
Figure 1
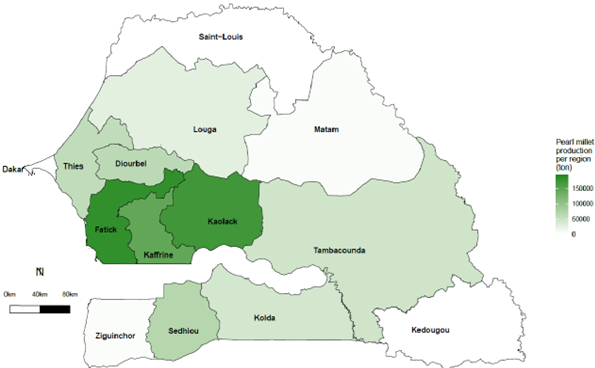
The total national production of pearl millet was 574,000 tons, which accounted for at least 33% of the total cereal production, behind only rice (44%) in 2018 (FAOSTAT, 2019). These ratios among different crops change over years depending on the financial support received for different grain crops (Figure 2A). Pearl millet has occupied the largest area harvested among the cereal crops, followed by rice, sorghum, and maize over the years (Figure 2B). However, the average grain yield remains very low and unstable, never reaching the threshold of 1000 kg ha-1 (Figure 2C). By comparison, pearl millet yields in India are 1,243 kg ha-1 for the same year (FAOSTAT, 2019). The adoption of hybrids has led to a significant improvement in productivity in India. The first public system for robust hybrid selection of pearl millet was established in the 1960s. This system has also been supported by the private sector and has facilitated significant improvement of productivity in the country with an average increase in grain yield of 24 kg/ha/year between 1996 and 2012 (Yadav & Rai, 2013). In 2006, over 70 hybrid varieties covered 60% of the cultivated area of pearl millet in India and these hybrid varieties were mainly developed by the private sector and consisted of more than 80% of varieties used at that time (Karandikar et al., 2018). In contrast, no hybrid variety was cultivated in Senegal.
Figure 2a
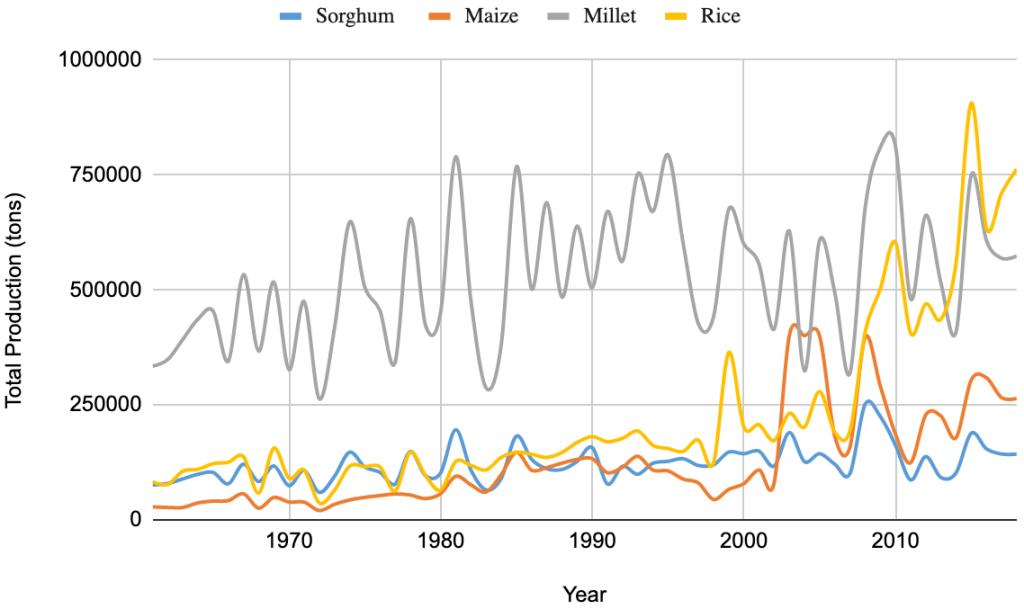
Figure 2b
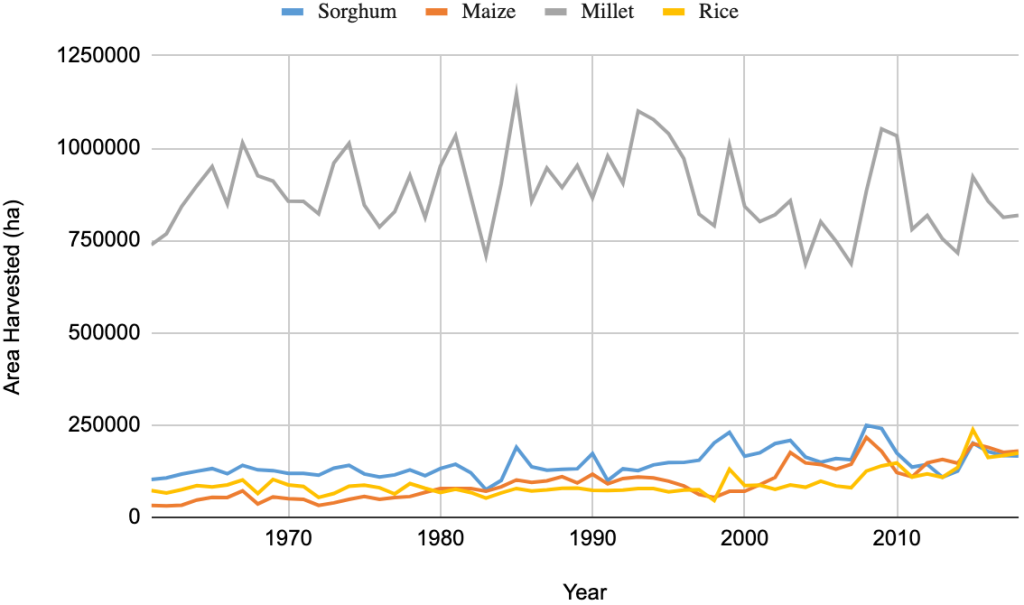
Figure 2c
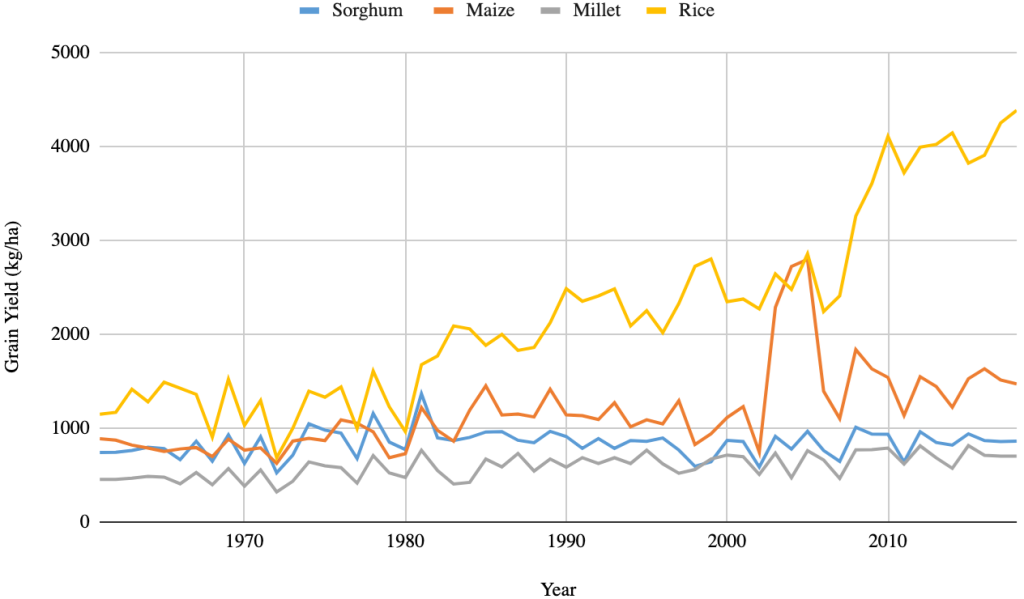
In addition to the absence of hybrid varieties in cultivated areas, growing unimproved varieties has been the major constraint of pearl millet grain productivity (Kanfany et al., 2020). In 2009, only a few improved varieties were cultivated in 34.5% of the national production area. The varieties Thialack 2, Souna 3, and IBMV 8402 covered only 16.5%, 14%, and 4% of the area, respectively (Walker, 2015). In addition, various biotic and abiotic stress conditions, including Striga weed (Striga hermonthica), low soil fertility, drought, and insect pests are limiting factors for yield. Moreover, lack of machinery for sowing, disease and bird damage, limited access to certified seeds of high-yielding varieties, and cultivable land all affect production of pearl millet in Senegal (Kanfany et al., 2020). Meanwhile, significant improvement has been made for rice and maize, with an increased productivity of 66% and 282%, respectively, mainly due to the use of higher-yielding varieties and pertinent crop management practices to alleviate biotic and abiotic stresses.
In recent years, the reduction in rainfall volume and the short period of rainfall during the growing seasons have negatively impacted the performance of pearl millet in Senegal (ANSD, 2020). As farmers usually plant pearl millet while waiting for or in response to the first rain of the year, the crop can face severe drought stress in early stages. It is proven that drought stress at an early stage significantly reduces the plant height, biomass, and total grain number and weight in pearl millet inbred lines (Debieu et al., 2018). Early ending of the rainy season can also lead to terminal drought episodes at the grain filling stage, especially for late- maturing varieties.
According to the current climate models used in Saharan Africa, including Senegal, more inter- and intra-annual variability in the rainfall amount and distribution is expected (Brown & Lall, 2006), increasingly threatening pearl millet productivity in the country. Several studies have predicted that changed climatic conditions in Sub-Saharan Africa will lead to significant and increasing yield losses for major crops by the 2050s if cropping systems do not adapt. The associated yield losses are forecast at 17% for millet (Schlenker & Lobell, 2010). The Groundnut Basin agro-ecological zone is the most important part of the national pearl millet production area, but it is characterized by a Sudano-Sahelian climate and will be particularly affected by this climate variability.
Past Attempts of Genetic Improvement and Achievements
1. Phase 1: Exploitation of the Local Germplasm for Grain Yield Improvement
The pearl millet improvement program started in Senegal in 1931 at the National Center for Agronomic Research (CNRA) in Bambey. There are two ecotypes of pearl millet traditionally grown in Senegal based on life cycle length: the short cycle ecotype (65–90 days) called ‘Souna’ and the longer cycle (120–150 days) called ‘Sanio’. Souna morphotypes flower from 50 to 60 days after planting and are adapted to ecosystems with low and irregular water supplies (350–600 mm annually rainfall). They represent 85% of total millet production and cover the most important part of the nationwide production area in the north and central regions (Diack et al., 2017). Sanio morphotypes flower from 80 to 110 days after planting and are mainly cultivated in the southern region, receiving more important rainfall (900–1200 mm).
During the first phase of the breeding program, the selection was mainly focused on producing inbred lines using pedigree selection from the two ecotypes that were collected locally across the country (Fofana, 1987). This approach led to the development of 113 inbred lines by 1949. However, due to the cross-pollinated nature of pearl millet, these lines lost vigor (Jones & Mangelsdorf, 1925). For instance, one of the local populations gave 20% more grain yield when compared to the performance of the inbred lines selected from this population (Fofana, 1987). Bono & Leclercq, 1963 realized that this breeding method could not be directly used since it only allowed the development of interesting, inbred lines. However, when the inbred lines were crossed with local populations, the top-cross hybrids produced up to 147% yield compared to the local population. By 1959, 28 inbred lines were selected and evaluated for overall performance in three consecutive years based on uniformity, yield performance, and yield component traits. The results showed that even if the homogeneity was significantly improved, the grain yield of these lines was not better than farmers’ cultivars (Fofana, 1987). Thus, pedigree selection breeding was judged as an unsuitable tool for pearl millet yield improvement.
In 1961, the national breeding program shifted from pedigree selection to recurrent selection using five local populations: three early (Souna) and two late (Sanio) pearl millet ecotypes (Etasse, 1965). The varieties, derived from the late populations, showed low performance in the first generation of recombination and selection was focused on the early maturing populations. The best progeny was selected from the three early populations and combined to create a synthetic variety named Souna 2. This variety showed high grain yield potential with a 107% and 121% yield increase compared to landraces in the first and second generation of recombination, respectively. However, the performance in farmer’s fields was still variable, mostly lower than local varieties in farmers’ fields (less than 2 t/ha). This variety was further improved by top-crossing with a local population (PC28)s using Souna 2 as the tester, resulting in the selection of eight elite lines from this population. The eight lines were combined in 1969 to form Souna 3, the first variety released by the breeding program (MAER, 2012). This synthetic variety was proposed for cultivation in the central and southeastern part of the Groundnut Basin agro-ecological zone (Table 1). This variety has a compact and cylindrical panicle, a resistance to smut and downy mildew, and a grain yield potential of 2.5 to 3.5 t ha-1 It has since been one of the most cultivated varieties in Senegal.
Variety Name | Type | Year of Creation | Year of Release | Maturity Cycle (days) | Potential Grain Yield (t ha-1) |
Souna 3 | Synthetic | 1969 | 1972 | 90 | 3.5 |
IBV 8001 | Synthetic | 1980 | 1987 | 80 | 3.4 |
IBV 8004 | Synthetic | 1980 | 1987 | 75 | 2.6 |
IBMV 8402 | Synthetic | 1984 | 1987 | 80 | 2.5 |
ISMI 9507 | Synthetic | 1995 | 2010 | 85 | 2.5 |
Gawane | Composite | 2006 | 2010 | 85 | 2.5 |
Thialack 2 | Composite | 2008 | 2010 | 95 | 3 |
Souna Sine | Composite | 2018 | 2021 | 90 | 3 |
Souna Saloum | Composite | 2018 | 2021 | 90 | 3 |
Souna Baol | Composite | 2018 | 2021 | 90 | 2.5 |
Taaw | Hybrid | 2017 | 2021 | 75 | 3.5 |
Note. Data compiled from ISRA’s variety fact sheets
2. Phase 2: Genetic Improvement of Harvest Index and Resistance to Drought
To achieve self-sufficiency through the improvement of harvest index in local populations, a multi-disciplinary pearl millet program involving several scientists was launched in 1970 (Fofana, 1987). This was one of the first initiatives to strengthen the breeding program after independence in 1960. Thus, 29 populations that were collected from the five main West African countries growing pearl millet were crossed with three dwarf parents sourced from the USA [23 D25 and 239 D2B and India (I 472)] (Fofana, 1987). This program developed two dwarf synthetic varieties, GAM 73 and GAM 75 (Table 1) from I 472 x HK 1133 and Tift 23 D2 x Aniata, respectively (Bilquez, 1975). These varieties performed well under irrigated and highly fertilized conditions but could not express their potential under regular farmers’ field conditions.
From the 1970s, Senegal experienced a period of severe drought that occurred throughout the entire Sahelian region, affecting crop production considerably (Nouaceur et al., 2017). In addition, the improved varieties in Senegal, as in most West African countries, were not widely diffused throughout the production area due to large environmental variations (Spillane & Gepts, 2001). The multidisciplinary team also developed early maturing (60 and 75 days) synthetic varieties, which were evaluated for overall performance from 1977 to 1979. However, these early varieties produced lower grain yield than Souna 3, even in the environments for which they were developed.
In 1974, the Senegalese Institute of Agricultural Research (ISRA) was established and started a new millet breeding program with the International Crops Research Institute for the Semi-Arid Tropics (ICRISAT) in 1977. This millet improvement program was funded by the United Nations Development Program (UNDP) with the main goal of diversifying the genetic bases to develop new varieties that combine stress tolerance and high grain yield potential. This effort made it possible to introduce several millet accessions from India. However, these accessions were not adapted to Senegalese growing conditions because they were matured too early,susceptible to diseases and insects, had small panicle size, and produced a similar grain yield as Souna 3. Besides the fact that the size of the panicle has been correlated with the grain yield, the length of the panicle is an important trait for the farmers related to the local practice during harvest. Indeed, the panicles are grouped and tied in heaps after harvest to facilitate transport.
Therefore, these accessions were crossed with local populations to generate new breeding materials, leading to the release of three varieties in 1987 (IBV 8001, IBV 8004, and IBMV 8402) to the central and northern part of the Groundnut Basin agro-ecological zone (Table 1). These varieties were early-maturing and tolerant to downy mildew (Gupta, 1986; Gupta & Ndoye, 1991). In order to boost the national pearl millet production, a small hybrid breeding program was initiated in 1982. Thus, several crosses were made between the selected inbred lines and the landraces with CMS lines introduced from ICRISAT in India. The best performing hybrid (ICMH 8413 from a cross of 81A x IBMI 8207) produced up to 50% more grain yield than Souna 3 (Ndoye & Gupta, 1987). However, these hybrids were not widely grown due to their downy mildew susceptibility, short panicle size, and dwarf stature as farmers preferred tall cultivars for complementary forage yield.
In the early 2000s, the West African National Agricultural Research Systems (NARS), ICRISAT, coordinated with Réseau Ouest et Centre Africain de Recherche sur le Mil (ROCAFREMI) and International Sorghum and Millet Collaborative Research Support Program (INTSORMIL) regional networks to characterize millet accessions collected from West and Central Africa (Haussmann et al., 2006). Selected accessions were evaluated in regional trials to identify specific varieties that performed well within a country and/or across different agro-ecological zones in West Africa (Wilson et al., 2008). In Senegal, ICTP-8203 and GB-8735 were identified for the Sahelian zone, ISMI-9301 and ISMI-9305 for Sudano-Sahelian zone, and SOSAT-C88 and ICMV-IS-88305 for the Sudanian zone. These varieties increased grain yield up to 22%, on average, compared to the previously released varieties.
3. Phase 3: Current Genetic Improvement of Pearl Millet in Senegal: A Decade of Rapid Progress
Since 2007, millet genetic improvement in the National Pearl Millet Program has been financially supported by the West Africa Agricultural Productivity Program (WAAPP), a 10-year program funded by the World Bank and several other international funding programs. These funds offered new opportunities to the national program. Significant efforts have been made by the millet program to assemble and define a national core collection, improve local populations, train young scientists. Progress has also been made on strengthening collaboration with National Agricultural Research Institutes and Advanced Research Centers for Germplasm Exchange and Evaluation, integrating new tools, such as molecular markers and high-throughput phenotyping with conventional breeding, and defining breeding product profile.
Genetic diversity of the germplasm used in a breeding program determines the potential genetic gain achievable through selection (Ambati & Singh, 2017). The national breeding program has made a particular effort to understand, conserve, exploit, and manage the available genetic resources across the country. The national pearl millet collection was updated in 2014 for better representation of the different varieties covering the entire national territory, especially the late flowering varieties (Diack et al., 2017). This new collection contained 477 accessions, including 353 early flowering (Souna), 112 late flowering (Sanio) and 12 improved varieties. These accessions showed high genetic diversity (He = 0.55). This consistently resulted in a greater genetic diversity in Senegalese landraces compared to other regions of Africa and Asia and was surely linked to the proximity of the center of domestication of the crop (Hu et al., 2015).
To facilitate the exploitation of all this diversity in the breeding program, a core collection was established based on phenotypic and genotypic data (SSR markers), using an advanced maximization strategy with a heuristic approach (Kim et al., 2007). This core collection consisted of 91 accessions (22% of total accessions), including 31 Sanio and 60 Souna, that captured as much genetic diversity as possible from the collected genetic material. The assessment of this core collection’s structure revealed two genetic pools (genetic differentiation between Sanio and Souna). Each pool was further divided into three phenotypic subgroups based on the targeted phenotypic traits, such as yield and flowering time. Phenotyping of this core collection revealed five traits, including two phenological traits (heading and flowering) and three morphological traits (biomass, plant height, and tillering) that could clearly differentiate the Souna ecotype from the Sanio ecotype (Diack et al., 2020).
These identified groups and subgroups constituted potential heterotic groups that could be exploited in the millet breeding program to improve the phenotypic traits associated with these differentiations. Heterotic groups are very important for hybrid breeding because they guide the choice of parental lines, optimize the use of genetic material, and maximize the performance of derived hybrids (Meena et al., 2017). The Senegalese breeding program recently initiated studies to identify and validate heterotic groups in the core collection for hybrid breeding as part of a USAID-funded project (Genetic Enhancement of Pearl Millet). This study identified the most representative genotypes of the core collection and produced F1s from crosses between these individuals. Evaluation of these F1s and their parents for general and specific combining ability is currently in progress.
As performance of hybrid lines can be predicted based on general combining ability, trait introgressions in recurrent selection schemes are more efficient using divergent heterotic groups than genetically similar heterotic groups (Reif et al., 2007). Evaluation of hybrids, generated by crossing 17 open-pollinated varieties (OPVs) in a diallel mating design in nine environments over two years in Niger and Senegal resulted in the identification of 5 potential parental lines (Nigerien CIVT, H80-10Gr, Taram and Senegalese Thialack 2, and Souna 3). These five parental lines have a high combining ability that can be used to initiate reciprocal recurrent selection (Sattler et al., 2019).
The combining ability and heterosis for grain yield and its associated traits (i.e., plant height, flowering time, panicle length and diameter, productive tillers, thousand grains weight, and panicle and downy mildew) were assessed by crossing 17 inbred lines with Sosat C88 and Souna 3. The results indicated that the inheritance of these traits was controlled by both additive and nonadditive gene effects although the contribution of the additive genes was greater (Kanfany et al., 2018a). Some inbred lines (IBL003-B-1, IBL091-1-1, IBL095-4-1, IBL110-B-1, and IBL206-1-1) showed positive General Combining Ability (GCA) effects on grain yield and negative GCA effects on downy mildew, flowering time, and plant height; therefore, they are good parents for breeding high-yielding open-pollinated varieties and hybrids (Kanfany et al., 2018b).
Crop breeding is now undergoing a paradigm shift with the development of modern approaches. These methods, such as marker assisted selection or genomic selection, are more accurate and less costly in time and effort and are a byproduct of next-generation sequencing (NGS). In an effort to understand the genomic diversity and population structure of Senegalese germplasm, all accessions in the core collection were characterized using Genotype By Sequencing (GBS). This yielded 21,663 polymorphic (biallelic) and high-quality Single Nucleotide Polymorphism (SNP) markers. Marker-trait association identified markers linked to the adaptive genes PgPHYC and PgMADS11 that were involved in the variation of flowering time in millet (Diack et al., 2017). Eighteen SNPs and genes have been associated with flowering time, plant height, number of tillers, and biomass, with some of these genes acting as candidate genes for abiotic stress adaptation. These identified markers, after validation, will be useful for marker-assisted selection in the program.
Cost and time efficiency in breeding programs depend on the ability to identify parental lines at an early stage of the breeding scheme (Ambati & Singh, 2017). To this end, studies were carried out to identify donor parents for the traits of farmers’ interest. Firstly, genotypes with desirable agronomic traits and stable resistance to downy mildew (DM) were identified in hotspot areas (Zoclanclounon et al., 2019). Then, inbred lines, derived from a collection of landraces originating from West and Central African countries, were evaluated in the Bambey and Nioro Research Stations under field conditions with spreader rows of DM, using SOSAT-C88 and 7042S as resistant and susceptible controls, respectively (Kanfany et al., 2018b). Highly significant differences were observed among 101 lines tested for DM incidence and severity as well as agro-morphological traits, including plant height, flowering time, panicle length, and productive tillers. Based on DM incidence and severity of control varieties used, the testing lines were grouped into three categories: resistant (55, including SOSAT-C88), moderately resistant (16), and susceptible (30, including 7042S). Also, using DM resistance and plant height as the most discriminant factors, hierarchical ascendant cluster analysis grouped these entries into three clusters, confirming the previous categorization.
Conversely, the released Senegalese varieties (Gawane, ISMI9507, Thialack2 varieties, IBV8004, and Souna 3) were evaluated for resistance to stem borers and maize head miner (MHM) under natural infestation in the 2014 and 2015 cropping seasons (Goudiaby et al., 2018). Gawane was identified to be tolerant to stem borers and Thialack2 and ISMI9507 were identified to be resistant to MHM (Goudiaby et al., 2018). This effort to identify new sources of resistance to several diseases under Senegalese agro-ecological conditions provides new genetic resources for improvement of pearl millet for agronomic traits and resistance to DM, MHM, and stem borer. These new resources will be used in the national breeding program to combine into new varieties resistant to biotic stresses and with desirable adaptation traits.
All these efforts allowed the breeding program to select and propose four new pearl millet varieties for release in 2021, including three OPV (SL28, SL169, and SL423) and one top-cross hybrid. The OPVs were derived from local populations, which were improved through recurrent selection using the S1 method. The top-cross hybrid named TAAW was created by crossing a local population (SL175) to a male sterile parent sourced from ICRISAT (ICMA-177090).
4. Productivity Improvement Over the Different Phases
The average rates of yield gains from each of the three phases of pearl millet improvement in Senegal are listed in Figure 3. In the first phase (1961–1973), low average grain yield was observed throughout the country, mainly due to drought stress in early stages and lack of adapted varieties (ANSD, 2020). Thus, the breeding efforts at that period were focused on the development of varieties adapted to specific agroclimatic zones. The breeding effort in the second phase (1974–2007) led to the release of several new varieties, with an increased yield of 2.06 kg/ha. The highest rate of productivity was achieved in the third phase (2008–2019), with a yield gain of 2.7 kg/ha/yr. However, the genetic gain through these three phases remains unknown.
Figure 3
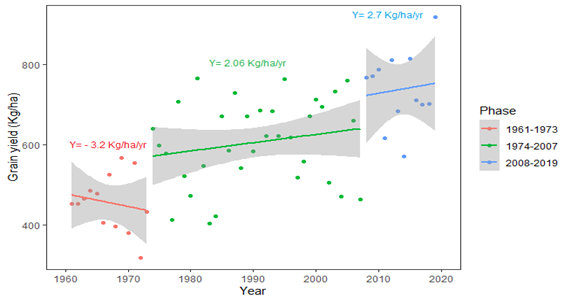
Assuming that this continued increase in average grain yield has been achieved through genetic gain, it promises great potential for further improvement in millet yield, especially with the help of newly developed genomic tools. However, it should be considered that this rapid increase in grain yield may be caused by the combination and fixation of favorable alleles at major effect loci that underlie traits positively correlated with grain yield. Indeed, selection has been shown to generally fix favorable alleles at major effect loci faster than those at minor effect loci (Mayo & Hancock, 1981). In this case, it would be expected that the yield improvement be less significant in the coming years since it would be mainly driven by the fixation of favorable alleles at loci with minor effects and would depend more heavily on better management practices (Mayo & Hancock, 1981; Xu et al., 2017; Yeaman, 2022). Nevertheless, the breeding history described above and the current breeding pipeline which regularly brings genetic material from the associated programs suggest that the large effect variants have not yet segregated into the breeding germplasm (Mayo & Hancock, 1981; Muleta et al., 2019). Therefore, more significant yield improvement through genetic improvement should reasonably be targeted.
Future Genetic Improvement Strategies
Grain yield is unanimously considered the most important trait by farmers (Kanfany et al., 2020). Drought tolerance, adaptation to low soil fertility, grain color, disease and insect resistance, and early maturity are also important traits to be considered when farmers select a pearl millet variety to grow in their fields (Kanfany et al., 2020). Therefore, these should be the target traits for developing a variety that grows in Senegalese conditions. However, the order of priority for these traits varies from location to location. Incorporating farmers’ preferred basic and value-added traits into the breeding product profile would certainly increase farmer acceptance of released varieties.
The breeding goal in Senegal is to release pearl millet varieties with high yields (15% increase in grain yield over Souna 3 and Thialack II), high iron contents (with > 40 ppm Fe), and better adaptation in the two agro-ecological zones (Table 2). The combined strategies described below should enable the National Pearl Millet Improvement Program to diversify its sources of germplasm and accelerate varietal development with better selection accuracy.
Breeding Targets | Target Agro-ecologies | Maturity (days) & Rainfall | Resistance/tolerance Required | Basic Traits | Value Added Traits | Breeding Goals |
High yielding, early maturing pearl millet OPVs for adaptation to Sahelian zone of Senegal | Target: Central and northern part of the peanut basin agro-ecological zone | Early maturity 70–90 days
(350–600 mm/annul) |
Biotic stresses: Downy mildew, millet head-miner Striga hermonthicaAbiotic stresses: Drought, Low soil fertility |
Grain Yield of >2 tons/ha, Downy mildew resistanceHead length > 40 cm |
High grain iron and zinc content. Striga tolerance Drought tolerance Head miner tolerance |
10% increase in grain yield over improved check (Souna 3) with >40 ppm Fe
|
Medium maturity, high yielding pearl millet OPV Soudanian zone of Senegal | Target: Southern part of the peanut basin agro-ecological zone | Medium maturity/ >90 days(500–900 mm/annum) |
Biotic stresses: Downy mildew, head miner, striga hermonthicaAbiotic stresses: Drought, low soil fertility |
Grain Yield of >2 tons/ha, downy mildew resistanceHead length > 50 cm |
High grain iron and zinc content.
Striga tolerance |
10% increase in grain yield over improved check (Thialack II) with >40 ppm Fe
|
Early maturity, high yielding hybrids for adaptation to better endowed environments of West Africa | Target: central and northern part of the peanut basin agro-ecological zone | Early 70–90 days /
(350–800 mm/annum) |
Biotic stresses: Downy mildew resistance, head minerAbiotic stresses: Drought, low soil fertility |
Grain yield of > 2.0 tons/ha Downy mildew resistanceHead length > 50 cm |
High grain iron and zinc content.
Striga tolerance Drought tolerance |
15% increase in grain yield over improved check (Souna 3) with >40 ppm Fe
|
I. Towards Implementing Gonomics-enabled Breeding
In the current breeding scheme, phenotypic selection remains the major method used in Senegal. However, this approach may not be able to properly examine the hidden genetic variation available for complex agronomic traits, such as grain yield and drought resistance, as these traits typically have low heritability and are heavily influenced by environments (Wu et al., 2012). Moreover, phenotypic selection involves many selection cycles in multi-trials in different environments to accurately evaluate progeny performance, which not only needs large amounts of seed for replicated trials, but is also laborious, time-consuming, and costly. However, such tests may not be realized due to insufficient seed amount and financial support for resource-limited breeding programs of developing countries like Senegal. Furthermore, the current and predicted variation in rainfall amount and distribution in the Sahel zone has emphasized the difficulty to control genotype x environment (GxE) interactions.
In light of the challenge of increasing genetic gain with limited resources, the National Pearl Millet Improvement Program has considered making greater use of currently available genomic resources to help breeders make more informed decisions about crosses at early breeding stages. The availability of the pearl millet reference genome sequence (~1.79 Gb) and the reduction in the cost of high-throughput data acquisition offers the opportunity to strengthen the breeding pipeline (Varshney et al., 2017). Nevertheless, the choice of modern breeding tools and methods to integrate must be evaluated with the utmost caution, considering several factors, including the genetic architecture of the targeted traits. For instance, the results of simulations performed by Muleta et al. (2019) showed that there are specific conditions under which genomics-assisted recurrent selection (GARS) should be considered by small breeding programs, according to the genetic architecture of the trait. In fact, this study showed a lower cost per unit gain using phenotypic recurrent selection (PRS) compared to GARS for oligogenic traits in small new breeding programs, while GARS gave lower cost per unit gain than PRS with larger populations and polygenic traits. Thus, understanding the genetic architecture of target traits will guide the breeding program in adopting the most effective breeding pipeline to maximize genetic gain given available resources.
Genomics-assisted breeding that integrates advanced genomic tools with conventional breeding has been one of the main strategies for developing high-yielding crop varieties that are resistant to biotic and abiotic stresses. Over the past decade, the National Pearl Millet Improvement Program has focused on understanding the genomic diversity of available germplasm, identifying genetic variants of targeted traits, and uncovering the molecular and physiological mechanisms underlying the expression of these traits. Phenotypic variants identified for downy mildew, MHM, and drought tolerance and allelic variants (SNPs) identified for flowering time provide new genetic resources for the development of molecular markers linked to favorable alleles. Therefore, more accurate selection for these traits could be achieved using marker-assisted backcrossing to shorten breeding cycles (Bernardo, 2016). In the years to come, the National Pearl Millet Improvement Program will take advantage of new financial resources from the Sorghum and Millet Innovation Lab (SMIL), Innovation Lab for Crop Improvement (ILCI), and Desira-ABEE to set up a strong marker-assisted breeding program to accelerate the introgression of these genetic variants into elite lines to improve the genetic gain.
2. Breeding for Drought Adaptive Traits in the Senegalese Agro-ecosystem
Drought tolerance is a polygenic trait significantly influenced by the environment (Serba & Yadav, 2016). The development of drought-tolerant varieties is a challenging task that can be achieved through the introgression of adaptive traits that allow plants to grow and produce satisfactory yields under water-limited conditions. In some cases, complex traits, such as drought, can be more efficiently improved by targeting simpler and more strongly correlated traits with them (Breseghello & Coelho, 2013). Drought adaptive traits can be categorized into three main strategies: drought escape, dehydration avoidance, and dehydration tolerance (Basu et al., 2016). Drought escape allows plants to accelerate their life cycle and reproduce before drought occurs (Turner, 1896). This strategy is commonly used by annual plants although the yield is generally decreased (Lisar et al., 2016). Dehydration avoidance is a strategy for preserving the high-water potential of plants mainly by reducing water loss through transpiration and optimizing water uptake from the soil by the root system (Basu et al., 2016). Because the mechanisms involved in this strategy consume additional energy, plant yield is generally decreased and the plants are small (Lisar et al., 2016). Avoidance is mainly achieved through traits related to root architecture, stomatal control, and transpiration efficiency (Basu et al., 2016). Dehydration tolerance involves mechanisms to maintain functionality in a water stressed environment (Turner, 1896). Dehydration tolerance involves traits such as cell remobilization of stem water-soluble carbohydrates, membrane stability and osmotic adjustment (Lisar et al., 2016). A drought tolerance cultivar can be defined as a genotype that detects water deficiency and effectively adopts combined strategies to grow and produce a satisfactory yield. Understanding the traditional cropping systems’ response to water deficiency and identifying the valuable traits to be targeted are critical steps in breeding a drought tolerant cultivar.
Through evolution, traditional pearl millet landraces showed evidence of different mechanisms of adaptation to climate change, resulting from natural selection as well as from farmers’ selection. On the one hand, the development of the root system of pearl millet is distinguished by a fast-growing primary root that rapidly colonizes the deeper horizons of the soil at an early stage (Passot et al., 2016). This trait allows access to the water available in a deeper soil horizon. Comparison of root development parameters in 16 inbred lines originating from Indian, West African, and Central African landraces showed significant variation in primary root length and lateral root density that could be exploited in subsequent breeding efforts (Passot et al., 2016). On the other hand, West African pearl millet farmers preferably cultivate high tillering landraces with small grains (Serba & Yadav, 2016). These landraces can produce secondary tillers and fill the grain very quickly. Since yield potential is related to the number of tillers and grains, among other morphological traits, this strategy allows them to efficiently improve yield under drought conditions. Finally, evidence is beginning to accumulate that flowering time is under selection in West Africa in response to rainfall variability. Flowering time directly correlates with annual rainfall (Saïdou et al., 2009).
Comparison analysis of pearl millet accessions collected in 1976 and 2003 in Niger showed a significant shift: varieties collected in 2003 flowered slightly earlier and had a shorter spike than in 1976 (Saïdou et al, 2009). This response to selection for flowering time was significantly associated with polymorphisms in two genes PHYC and PgMADS11 (Mariac et al., 2011; Saïdou et al., 2009). These polymorphisms for early and late flowering alleles in these two genes were found in the landraces in Niger and were also more recently revealed in the Senegalese germplasm (Diack et al., 2020; Vigouroux et al., 2011). These findings suggest that drought escape is a main mechanism of adaptation to drought in West Africa. Understanding the genetic basis of these morpho-physiological traits associated with drought tolerance, in particular the flowering period, and then identifying the favorable alleles to introgress into the elite background are promising steps for the rapid selection of a drought-tolerant cultivar.
3. Reinforcement of Hybrid Breeding Strategies
In view of the urgent need for a rapid increase in productivity, it is crucial to establish a strong hybrid breeding program to focus on breeding high-yielding hybrid cultivars. Combining ability studies have clearly highlighted the prevalence of high levels of heterosis and potential to breed for hybrids with high and stable yield, as well as multiple disease resistance and abiotic stress tolerance in Senegalese environments. Previous studies showed the superiority of hybrid varieties over the best landraces for agro-morphological and agronomic traits in West African pearl millet germplasm (Pucher et al., 2016).
Inspired by the success of hybrids in India, a collaboration has been initiated by millet breeding programs in several West African countries (Senegal, Niger, Burkina Faso, and Mali) as part of the SMIL project for the development of dual-purpose pearl millet hybrid lines with enhanced grain nutritional quality and stover digestibility. The aim of the SMIL project was to characterize genetic diversity, validate superior germplasm accessions, and identify genetic variants for traits related to stover quality and digestibility (i.e., grain mineral content, grain and stover yields) using 100 germplasm accessions collected from the related countries (Serba et al., 2020). Adoption of dual-purpose pearl millet hybrid varieties are expected to improve nutritional security of smallholder farming families, crop-livestock integration, and income generation.
Conclusion
Pearl millet is the main cereal grown in Senegal. The traditional farming system has played a crucial role in creating and maintaining a high diversity among landraces. The Senegalese breeding program has invested a great deal of effort for nearly a century in collecting, maintaining, and more recently understanding this genetic diversity. In parallel, several varieties were derived from the local population using the conventional breeding approach. The regional and international partnerships developed have made it possible to enrich the genetic material and support all these selection efforts, resulting in a significant improvement in yield performance, especially in the last phase ranging from 2008–2019. This last decade was particularly marked by progress towards the implementation of genomics-based breeding and the strengthening of hybrid breeding strategies. Despite their yield performance, the varieties released so far do not seem to hold all the agro-morphological characteristics that lead to their high adoption by farmers. The high genetic variation found in Senegalese landraces that possess adaptive traits to the local environment, as well as traits preferred by farmers, is a promising resource for developing high-yielding crop varieties that are resistant to specific biotic and abiotic stresses. In addition, the future breeding strategies outlined in this chapter, and the more participatory breeding approach should enable the breeding program to improve genetic gain more effectively for farmers’ priority traits. Further, they will contribute to addressing the challenge of sustainably improving pearl millet productivity for food security and income generation in Senegal.
Acknowledgements
This study is made possible by the support of the American People provided to the Feed the Future Innovation Lab for Collaborative Research on Sorghum and Millet through the United States Agency for International Development (USAID) under Cooperative Agreement No. AID-OAA-A13-00047. The contents are the sole responsibility of the authors and do not necessarily reflect the views of USAID or the United States Government.
References
Ambati, S. & Singh, T. V. J. (2017). Heterotic grouping and its importance in tropical hybrid rice breeding. International Journal of Multidisciplinary Advanced Research Trends, 9(1), 179–187.
ANSD. (2018). Sénégal : Enquête démographique et de santé continue (EDS-Continue) 2017. Agence Nationale de la Statistique et de la Démographie (ANSD).
ANSD. (2020). Situation économique et sociale du Sénégal. Agence Nationale de la Statistique et de la Démographie (ANSD).
Basu, S., Ramegowda, V., Kumar, A., & Pereira, A. (2016). Plant adaptation to drought stress. F1000 Research 5, F1000 Faculty Rev-1554. https://doi.org/10.12688/f1000research.7678.1
Bernardo, R. (2016). Genomewide predictions for backcrossing a quantitative trait from an xxotic to an adapted line. Crop Science, 56, 1067–1075. https://doi.org/10.2135/cropsci2015.09.0586
Bilquez, A. F. (1975). Amélioration des mils au Sénégal: synthèse des résultats obtenus au cours des quatre premières années de travail et conclusions générales. I.S.R.A., 57 p. multigr.
Bono, M., & Leclercq, P. (1963). Méthodes d’amélioration variétale des mils et sorghos utilisées au CRA Bambey. L’Agronomie Tropicale. Série 1, Riz et Riziculture et Cultures Vivrières Tropicales, 18(1), 33–52. https://agritrop.cirad.fr/444782/
Breseghello, F., & Coelho, A. S. G. (2013). Traditional and modern plant breeding methods with examples in rice (Oryza sativa L.). Journal of agricultural and food chemistry, 61(35), 8277–8286.
Brown, C., & Lall, U. (2006). Water and economic development: The role of variability and a framework for resilience. Natural Resources Forum, 30(4), 306–317. https://doi.org/10.1111/j.1477-8947.2006.00118.x
Burgarella, C., Cubry, P., Kane, N. A., Varshney, R. K., Mariac, C., Liu, X., Shi, C., Thudi, M., Courdec, M., Xu, X., Chitikineni, A., Scarcelli, N., Barnaud, A., Rhoné, B., Dupuy, C., François, O., Berthouly-Salazar, C., & Vigouroux, Y. (2018). A western Sahara centre of domestication inferred from pearl millet genomes. Nature ecology & evolution, 2(9), 1377-1380.https://doi.org/10.1038/s41559-018-0643-y
Debieu, M., Sine, B., Passot, S., Grondin, A., Akata, E., Gangashetty, P., Vadez, V., Gantet, P., Foncéka, D., Cournac, L., Hash, C. T., Kane, N. A., Vigouroux, Y., & Laplaze, L. (2018). Response to early drought stress and identification of QTLs controlling biomass production under drought in pearl millet. PLoS One, 13(10), https://doi.org/10.1371/journal.pone.0201635
Diack, O., Kane, N. A., Berthouly-Salazar, C., Gueye, M. C., Diop, B. M., Fofana, A., Sy, O., Tall, H., Zekraoui, L., Piquet, M., Couderc, M., Vigouroux, Y., Diouf, D., & Barnaud, A. (2017). New genetic insights into pearl millet diversity as revealed by characterization of early-and late-flowering landraces from Senegal. Frontiers in plant science, 8(818). https://doi.org/10.3389/fpls.2017.00818
Diack, O., Kanfany, G., Gueye, M. C., Sy, O., Fofana, A., Tall, H., Serba, D. D., Zekraoui, L., Berthouly-Salazar, C., Vigourouz, Y., Diouf, D., & Kane, N. A. (2020). GWAS unveils features between early-and late-flowering pearl millets. BMC Genomics, 21(1), 1–11.
Dussert, Y., Snirc, A., & Robert, T. (2015). Inference of domestication history and differentiation between early- and late-flowering varieties in pearl millet. Molecular Ecology, 24(7), 1387–1402. https://doi.org/10.1111/mec.13119
Etasse, C. (1965). Amélioration du mil Pennisetum au Sénégal. L’Agronomie Tropicale. Série 1, Riz et Riziculture et Cultures Vivrières Tropicales. https://agritrop.cirad.fr/435118/
FAOSTAT. (2019). Food and Agriculture Organization of the United Nations. FAO Database. Statistiques Agricoles. http://faostat.fao.org.
Fofana, A. (1987). Amélioration du mil au Sénégal. Annual report. Centre Nationale de Recherches Agronomiques. Bambey, Senegal. ISRA, CNRA.
Goudiaby, M. F., Sarr, I., & Sembene, M. (2018). Source of resistance in pearl millet varieties against stem borers and the ear headminer. Journal of Entomology and Zoology Studies, 6(1), 1702–1708.
Gupta, S. (1986). The pearl millet improvement program in Senegal. Annual Report. Centre Nationale de Recherches Agronomiques, Bambey, Senegal. ICRISAT, ISRA, UNDP, Cooperative Programme 1977–1985.
Gupta, S. C., & Ndoye, A. T. (1991). Yield stability analysis of promising pearl millet genotypes in Senegal. Maydica, 36(1), 83–86.
Haussmann, B. I. G., Boubacar, A., Boureima, S. S., & Vigouroux, Y. (2006). Multiplication and preliminary characterization of West and Central African pearl millet landraces. International Sorghum and Millets Newsletter, 47, 110–112.
Hu, Z., Mbacké, B., Perumal, R., Guèye, M. C., Sy, O., Bouchet, S., Prasad, P. V. V., & Morris, G. P. (2015). Population genomics of pearl millet (Pennisetum glaucum (L.) R. Br.): Comparative analysis of global accessions and Senegalese landraces. BMC Genomics, 16(1), 1–12.
Jones, D F, & P. C. Mangelsdorf. (1925). The improvement of naturally cross-pollinated plants by selection in self-fertilized lines: The production of inbred strains of corn. Bulletin, Connecticut Agricultural Experiment Station, 266, 349–418.
Kanfany, G., Diack, O., Kane, N. A., Gangashetty, P. I., Sy, O., Fofana, A., & Cisse, N. (2020). Implications of farmer perceived production constraints and varietal preferences to pearl millet breeding in Senegal. African Crop Science Journal, 28(3), 411–420. https://doi.org/10.4314/acsj.v28i3.6
Kanfany, G., Fofana, A., Tongoona, P., Danquah, A., Offei, S., Danquah, E., & Cisse, N. (2018a). Estimates of combining ability and heterosis for yield and its related traits in pearl millet inbred lines under downy mildew prevalent areas of Senegal. International Journal of Agronomy. https://doi.org/10.1155/2018/3439090
Kanfany, G., Fofana, A., Tongoona, P., Danquah, A., Offei, S., Danquah, E., & Cisse, N. (2018b). Identification of new sources of resistance for pearl millet downy mildew disease under field conditions. Plant Genetic Resources, 16(4), 397–400.
Karandikar, B., Smale, M., Birol, E., & Tedla-Diressie, M. (2018). India’s pearl millet seed industry: Prospects for high-iron hybrids. Harvest. Work. Pap., 28, 1–41.
Kim, K. W., Chung, H. K., Cho, G. T., Ma, K. H., Chandrabalan, D., Gwag, J. G., Kim, T. S., Cho, E. G., & Park, Y. J. (2007). PowerCore: a program applying the advanced M strategy with a heuristic search for establishing core sets. Bioinformatics, 23(16), 2155–2162. https://doi.org/10.1093/bioinformatics/btm313
MAER, Ministère de l’Agriculture et de l’Equipement Rural. (2012). Catalogue officiel des espèces et des variétés cultivées au Sénégal. Dakar, Senegal.
Manning, K., Pelling, R., Higham, T., Schwenniger, J. L., & Fuller., D. Q. (2011). 4500-year old domesticated pearl millet (Pennisetum glaucum) from the Tilemsi Valley, Mali: new insights into an alternative cereal domestication Pathway. Journal of Archaeological Science 38(2), 312–322. https://doi.org/10.1016/j.jas.2010.09.007
Mariac, C., Jehin, L., Saïdou, A. A., Thuillet, A. C., Couderc, M., Sire, P., Jugdé, H., Adam, H., Bezançon, G., Pham, J. L., & Vigouroux, Y. (2011). Genetic basis of pearl millet adaptation along an environmental gradient investigated by a combination of genome scan and association mapping. Molecular Ecology, 20(1), 80–91. https://doi.org/10.1111/j.1365-294X.2010.04893.x
Mayo, O., & Hancock, T. W. (1981). Fixation of genes having large or small effects on a trait with an intermediate optimum. Human Heredity, 31(5), 286–290. https://doi.org/10.1159/000153224
Meena, A. K., Gurjar, D., Patil, S. S., & Kumhar, B. L. (2017). Concept of heterotic group and its exploitation in hybrid breeding. International Journal of Current Microbiology and Applied Sciences, 6(6), 61–73. https://doi.org/10.20546/ijcmas.2017.606.007
Muleta, K. T., Pressoir, G., & Morris, G. P. (2019). Optimizing genomic selection for a sorghum breeding program in Haiti: A simulation study. G3: Genes, Genomes, Genetics, 9(2), 391–401. https://doi.org/10.1534/g3.118.200932
Ndoye, A. T., & Gupta, S. C. (1987). Research on pearl millet hybrids in Senegal in the international crops research institute for the semi-arid. Proceedings of the international pearl millet workshop. ISRA/ICRISAT.
Nouaceur, Z., Murărescu, O., & Murătoreanu, G. (2017). Rainfall variability and trend analysis of multi annual rainfall in Romanian plain. Annals of Valahia University of Targoviste, Geographical Series, 17(2), 124–144.
Oumar, I., Mariac, C., Pham, J. L., & Vigouroux, Y. (2008). Phylogeny and origin of pearl millet (Pennisetum glaucum [L.] R. Br) as revealed by microsatellite loci. Theoretical and Applied Genetics, 117(4), 489-497.
Passot, S., Gnacko, F., Moukouanga, D., Lucas, M., Guyomarc’h, S., Ortega, B. M., Atkinson, J. A., Belko, M. N., Bennett, M. J., Gantet, P., Wells, D. M., Guédon, Y., Vigouroux, Y., Verdeil, J. L., Muller, B., & Laplaze, L. (2016). Characterization of pearl millet root architecture and anatomy reveals three types of lateral roots. Frontiers in Plant Science, 7, 829. https://doi.org/10.3389/fpls.2016.00829
Pucher, A., Sy, O., Sanogo, M. D., Angarawai, I. I., Zangre, R., Ouedraogo, M., Boureima, S., Hash, C. T., & Haussmann, B. I. (2016). Combining ability patterns among West African pearl millet landraces and prospects for pearl millet hybrid breeding. Field Crops Research, 195, 9–20. https://doi.org/10.1016/j.fcr.2016.04.035
Reif, J. C., Gumpert, F. M., Fischer, S., & Melchinger, A. E. (2007). Impact of interpopulation divergence on additive and dominance variance in hybrid populations. Genetics, 176(3), 1931–1934. https://doi.org/10.1534/genetics.107.074146
Saïdou, A. A., Mariac, C., Luong, V., Pham, J. L., Bezançon, G., & Vigouroux, Y. (2009). Association studies identify natural variation at PHYC linked to flowering time and morphological variation in pearl millet. Genetics, 182(3), 899–910.
Saleh, A. S. M., Zhang, Q., Chen, J., & Shen, Q. (2013). Millet grains: Nutritional quality, processing, and potential health benefits. Comprehensive Reviews in Food Science and Food Safety, 12(3), 281–295. https://doi.org/10.1111/1541-4337.12012
Salehi-Lisar, S. Y., & Bakhshayeshan-Agdam, H. (2016). Drought stress in plants: causes, consequences, and tolerance. In Drought Stress Tolerance in Plants, Vol 1 (pp. 1-16). Springer, Cham.
Sattler, F. T., Pucher, A., Kassari Ango, I., Sy, O., Ahmadou, I., Hash, C. T., & Haussmann, B. I. (2019). Identification of combining ability patterns for pearl millet hybrid breeding in West Africa. Crop Science, 59(4), 1590–1603. https://doi.org/10.2135/cropsci2018.12.0727
Schlenker, W., & Lobell, D. B. (2010). Robust negative impacts of climate change on African agriculture. Environmental Research Letters, 5(1).
Sehgal, D., Skot, L., Singh, R., Srivastava, R. K., Das, S. P., Taunk, J., Sharma, P. C., Pal, R., Raj, B., Hash, C. T., & Yadav, R. S. (2015). Exploring potential of pearl millet germplasm association panel for association mapping of drought tolerance traits. PLoS One, 10(5). https://doi.org/10.1371/journal.pone.0122165
Serba, D. D., Muleta, K. T., St. Amand, P., Bernardo, A., Bai, G., Perumal, R., & Bashir, E. (2019). Genetic diversity, population structure, and linkage disequilibrium of pearl millet. The Plant Genome, 12(3). https://doi.org/10.3835/plantgenome2018.11.0091
Serba, D. D., Perumal, R., Tesso, T. T., & Min, D. (2017). Status of global pearl millet breeding programs and the way forward. Crop Science, 57(6), 2891–2905. https://doi.org/10.2135/cropsci2016.11.0936
Serba, D. D., Sy, O., Sanogo, M. D., Issaka, A., Ouedraogo, M., Ango, I. K., Drabo, I., & Kanfany, G. (2020). Performance of dual-purpose pearl millet genotypes in West Africa: Importance of morphology and phenology. African Crop Science Journal, 28(4), 481–498.
Serba, D. D., & Yadav, R. S. (2016). Genomic tools in pearl millet breeding for drought tolerance: Status and prospects. Frontiers in Plant Science, 7, 1724. https://doi.org/10.3389/fpls.2016.01724
Spillane, C., & Gepts, P. (2001). Broadening the genetic base of crops: An overview. In H. D. Cooper, C. Spillane, & T. Hodgkin (Eds.), Broadening the genetic of crop production, (pp. 1–23). CABI Publishing.
Turner, N. C. (1986). Adaptation to water deficits: a changing perspective. Functional Plant Biology, 13(1), 175-190.
Varshney, R. K., Shi, C., Thudi, M., Mariac, C., Wallace, J., Qi, P., … & Xu, X. (2017). Pearl millet genome sequence provides a resource to improve agronomic traits in arid environments. Nature biotechnology, 35(10), 969-976.
Vigouroux, Y., Mariac, C., De Mita, S., Pham, J. L., Gérard, B., Kapran, I., Sagnard, F., Deu, M., Chantereau, J., Ali, A., Ndjeunga, J., Luong, V., Thuillet, A. C., Saïdou, A. A., & Bezançon, G. (2011). Selection for earlier flowering crop associated with climatic variations in the Sahel. PloS One, 6(5), https://doi.org/10.1371/journal.pone.0019563
Walker, T. S., & Alwang, J. (Eds.). (2015). Crop improvement, adoption, and impact of improved varieties in food crops in Sub-Saharan Africa. CABI Publishing.
Wilson, J. P., Sanogo, M. D., Nutsugah, S. K., Angarawai, I., Fofana, A., Traore, H., Ahmadou, I., & Muuka, F. P. (2008). Evaluation of pearl millet for yield and downy mildew resistance across seven countries in Sub-Saharan Africa. African Journal of Agricultural Research 3(4), 371–378.
Wu, X., Chang, X., & Jing, R. (2012). Genetic insight into yield-associated traits of wheat grown in multiple rain-fed environments. PloS One, 7(2), https://doi.org/10.1371/journal.pone.0031249
Xu, Y., Li, P., Zou, C., Lu, Y., Xie, C., Zhang, X., Prasanna, B., & Olsen, M. S. (2017). Enhancing genetic gain in the era of molecular breeding. Journal of Experimental Botany, 68(11), 2641-2666.
Yadav, O. P., & Rai, K. N. (2013). Genetic improvement of pearl millet in India. Agricultural Research, 2(4), 275–292.
Yeaman, S. (2022). Evolution of polygenic traits under global vs local adaptation. Genetics, 220(1), iyab134.
Zoclanclounon, Y. A. B., Kanfany, G., Kane, A., Foncéka, D., Ehemba, G. L., & Ly, F. (2019). Current status of pearl millet downy mildew prevalence across agroecological zones of Senegal. The Scientific World Journal, 2019. https://doi.org/10.1155/2019/1252653