5 IoT Insecurities of Things (Murthy)
“When wireless is perfectly applied, the whole earth will be converted into a huge brain, which, in fact, it is, all things being particles of a real and rhythmic whole. We shall be able to communicate with one another instantly, irrespective of distance. Not only this, but through television and telephony, we shall see and hear one another as perfectly as though we were face to face, despite intervening distances of thousands of miles, and the instruments through which we shall be able to do this will be amazingly simple compared with our present telephone. A man will be able to carry one in his vest pocket.”
Nicola Tesla [1]
Introduction
The rapid evolution of technology has reshaped our world in profound ways, with the advent of the Internet of Things (IoT) standing as a testament to the transformative power of innovation. From smart homes and cities to wearables, industrial automation, and healthcare, IoT technologies have permeated nearly every aspect of our daily lives, offering unparalleled connectivity, efficiency, and convenience. However, amidst the promises of this digital revolution, there exists a critical imperative to balance technological advancement with humanitarian and human-centric concerns. Throughout the chapter, we will use incidents from the past decade to highlight these challenges.
To understand the current landscape of IoT and its intersection with humanitarianism, it is instructive to trace the evolution of this transformative technology (Atzori et al., 2010, 2017; S. Li et al., 2015). The roots of IoT can be traced back to the early 1980s when devices and objects were connected to the Internet for data exchange and remote control. The idea of connecting devices and objects to the Internet to enable data exchange and remote control was initially explored in various forms. One of the early precursors to IoT was the development of networked vending machines in the 1980s, which allowed remote monitoring and management of inventory levels. Another significant milestone was the invention of RFID (Radio Frequency Identification) technology, which enabled the identification and tracking of objects wirelessly.
However, it was not until the late 1990s that the term “Internet of Things” was coined, heralding a new era of interconnectedness and digital transformation. In 1999, Kevin Ashton, a British technologist, first used the term “Internet of Things” while working at Procter & Gamble(Kramp et al., 2013). He used it to describe a system where objects could be uniquely identified and connected to the Internet for tracking and monitoring purposes. Since then, the term has gained traction and become widely used to describe the growing network of interconnected devices and sensors (see, for example (Krotov, 2017), (Feki et al., 2013),(Čolaković & Hadžialić, 2018)).
Today, IoT encompasses a vast ecosystem of interconnected devices and systems, revolutionizing industries, enhancing efficiency, and empowering individuals worldwide. This interconnected network spans multiple domains (see, for example (Babun et al., 2021; Lin et al., 2017; Miraz et al., 2015; Sobin, 2020)), each benefiting significantly from the capabilities of IoT technologies as illustrated in Figure 5-1.
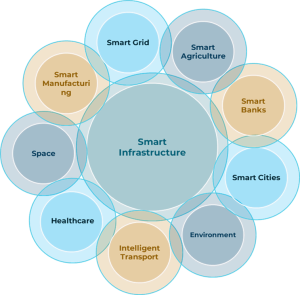
Smart Infrastructure serves as the backbone of modern intelligent systems. They integrate multiple technologies, including telecommunications, electrical energy, computing, and cognition. It provides the essential framework that supports the seamless operations and interconnection of various innovative domains. This infrastructure ensures that entities can connect, collect, process, and utilize resources made available in the various connected entities to potentially impact human life, livelihood, and their environment positively.
Table 5-1: IoT Domains v. Smart Infrastructure
Representative Domain | Domain Description | Need for Smart Infrastructure |
Smart Grid | Utilizes IoT to monitor and manage electricity distribution in real-time, integrating renewable energy sources and improving reliability.
(See, for example, (Abir et al., 2021; Pramudhita et al., 2018),(Kirmani et al., 2023)) |
Enables signal /data collection and analysis, optimizing electricity distribution, reducing outages, and facilitating the integration of various energy sources for efficient energy use and sustainability. |
Smart Manufacturing | It involves the use of connected machines and sensors to optimize production processes through real-time data analysis and predictive maintenance.
(See for example, (Soori et al., 2023),(Kusiak, 2018; Tao et al., 2018),(Yang et al., 2019)) |
Supports automation, monitoring, and adaptive control of production processes, leading to increased productivity, reduced downtime, and enhanced product quality. |
Smart Agriculture | It uses IoT devices and sensors to monitor soil conditions, weather patterns, and crop health, enabling precise farming practices.
(See, for example, (Abbasi et al., 2019),(Friha et al., 2021; Rajak et al., 2023; A. K. Singh, 2022; N. Singh & Singh, 2020)) |
Allows for precise resource management, optimizing irrigation, fertilization, and pest control, thereby increasing crop yields and promoting sustainable farming practices. |
Smart Banks | Transforms banking through enhanced security measures, personalized customer experiences, and streamlined operations with IoT-enabled devices and systems.
(See for example, (Khanboubi et al., 2019),(Lande et al., 2018),(B. Li et al., 2021),(Putra, 2021)) |
Enhances security, supports close to real-time fraud detection, and optimizes operational efficiency, providing more personalized and reliable banking services. |
Smart Cities | Integrates urban services such as transportation, utilities, waste management, and public safety through IoT. Sensors and connected devices provide real-time data to manage and improve city operations.
(See, for example, (Shih et al., 2016),(Rejeb et al., 2022),(Alavi et al., 2018),(Sanchez et al., 2014),(Zanella et al., 2014)) |
Enables efficient management of urban resources and services, reducing traffic congestion, lowering energy consumption, enhancing public safety, and improving the quality of urban life. |
Space | Supports space exploration and satellite operations through remote monitoring and control enabled by IoT. Provides critical data for scientific research and global communications.
(See also (Kua et al., 2021),(De Sanctis et al., 2016),(Qu et al., 2017),(Ray, 2022)) |
Supports precise monitoring and control of satellites and spacecraft, enhancing the efficiency and safety of space missions and facilitating advancements in space technology and exploration. |
Digital Healthcare | Includes remote patient monitoring, telemedicine, and innovative medical devices that collect health data and enable real-time monitoring and personalized treatment plans.
(See, for example, (Baker et al., 2017),(Javaid & Khan, 2021),(Farahani et al., 2018),(Al-rawashdeh et al., 2022),(Kelly et al., 2020)) |
Enables close to real-time health monitoring, early detection of health issues, and personalized treatment plans, improving patient outcomes and reducing healthcare costs. |
Intelligent Transport | Optimizes transportation systems with connected vehicles, intelligent traffic management, and real-time route planning through IoT. Integrated sensors provide data to improve logistics and public transit efficiency.
(See, for example, (Ushakov et al., 2022),(Zantalis et al., 2019),(Derawi et al., 2020),(Gerla et al., 2014),(Bellavista et al., 2013)) |
Supports efficient traffic management, reduces congestion, improves public transit services, and enhances overall transportation efficiency and safety. |
Environment | Monitors environmental factors such as air and water quality, pollution levels, and wildlife habitats using IoT sensors. Provides real-time data to address environmental challenges and promote sustainability.
(See, for example, (Anagnostopoulos et al., 2017),(Almalki et al., 2023),(Abegaz et al., 2018),(Singhvi et al., 2019),(Minoli et al., 2017)) |
Enables precise environmental monitoring, helping to mitigate pollution, manage natural resources sustainably, and respond effectively to environmental challenges. |
Source: Author
The interconnectedness and complexity of the representative domains and smart infrastructure showcase the need to consider IoT systems as significant, complex distributed systems that potentially make up the paradigms of systems of systems and networks of networks. This perspective is essential for understanding how these systems function and thrive across various domains.
A system of systems (SoS) is an assemblage of multiple independent systems that integrate and work together to achieve a higher level of functionality and performance than the individual systems could achieve alone. In our view, SoS has three essential characteristics – independence, collaboration, and complexity. Each constituent system operates independently and can fulfill its purpose even when detached from the SoS. The systems collaborate and interact, sharing data and resources to achieve common goals. The interactions and interdependencies among the systems create a complex structure that is greater than the sum of its parts (see, for example,(Maier, 1996),(Ren, 2017)).
IoT systems are composed of multiple semi-autonomous subsystems that must coordinate their actions to achieve overall system goals. For instance, in an intelligent city, the traffic management system, public safety system, and utility services must work together seamlessly. This interdependency requires a robust infrastructure that supports communication and data exchange among subsystems. Each subsystem in an IoT network can perform its tasks independently, but when integrated, they provide enhanced capabilities. For example, integrating IoT in healthcare with smart home devices can enable continuous patient monitoring and automated emergency responses, improving patient care beyond what each system could achieve individually. Treating IoT systems as SoS allows for scalable and flexible solutions. New subsystems can be added without redesigning the entire system, which is crucial for adapting to evolving technological advancements and increasing demands. This modular approach ensures that systems can grow and develop.
A Network of Networks (NoN) refers to multiple, interconnected networks that combine to form a more extensive, more complex network (Gao et al., 2014). This structure allows for extensive connectivity and data exchange across different domains and applications. Like our SoS perspective, it has three essential characteristics – scalability, diversity, and resilience. NoN can scale by integrating additional networks without disrupting the overall functionality. It includes various types of networks (e.g., wireless, wired, sensor networks) that serve different purposes but interconnect to provide comprehensive coverage and connectivity. The interconnected nature provides redundancy, enhancing the system’s resilience to failures.
IoT systems rely on the interconnection of multiple networks, including local sensor networks, communication networks, and cloud networks. This interconnectedness ensures robust connectivity, enabling continuous data flow and real-time responses. For example, smart agriculture relies on local sensor networks in fields connected to cloud platforms for data analytics and decision-making. Different IoT applications require various communication protocols to meet their specific needs, such as low latency, high bandwidth, or long-range communication. They view IoT systems as NoN, which allows for the integration of multiple communication technologies, such as Zigbee, LoRa, and 5G, to ensure that each application can operate optimally. By interconnecting various networks, IoT systems can achieve higher resilience and redundancy. If one network fails, the system can reroute data through another network, ensuring continuous operation. This is particularly important in critical applications like smart grids and intelligent transportation systems, where downtime can have significant consequences.
The global IoT ecosystem itself is a network of networks encompassing various domains like smart homes, industrial IoT, healthcare, and environmental monitoring, all interconnected through the Internet and other communication protocols.
Viewing IoT systems as systems of systems and networks of networks is crucial for understanding their complexity and potential. This perspective helps in designing robust, scalable, and flexible solutions that can adapt to diverse and evolving needs across various domains. It emphasizes the importance of interconnectivity, interoperability, and coordinated functioning of multiple subsystems, enabling IoT systems to deliver enhanced capabilities and improved outcomes in areas such as smart cities, healthcare, agriculture, manufacturing, and more.
Defining IoT
We consider the Internet of Things as a vast ecosystem of interconnected devices and systems that communicate and exchange data over networks to perform a variety of tasks, improve efficiency, and enhance decision-making across different domains. IoT systems are characterized by their ability to integrate multiple independent systems (SoS) and connect diverse networks (NoN) to function as cohesive, intelligent environments. The Internet of Things (IoT) is a multifaceted concept with varying definitions that emphasize different scopes, characteristics, and technological aspects. The 2010s have seen increasing use of “things,” though the definition had changed from when it started. There appears to be a diversity of definitions, with each meaning different, particularly in the proliferation of cloud platforms.
IEEE Internet Initiative [2] (Minerva et al., 2015) defined IoT as, “Internet of Things envisions a self-configuring, adaptive, complex network that interconnects ‘things’ to the Internet through the use of standard communication protocols. The interconnected things have physical or virtual representation in the digital world, sensing/actuation capability, and a programmability feature, and are uniquely identifiable. The representation contains information including the thing’s identity, status, location, or any other business, social, or privately relevant information. The things offer services, with or without human intervention, through the exploitation of unique identification, data capture and communication, and actuation capability. The service is exploited through the use of intelligent interfaces and is made available anywhere, anytime, and for anything, considering security.” While ITU(Y.2060: Overview of the Internet of Things, n.d.) has defined IoT as, “The Internet of Things (IoT) is a global infrastructure for the information society, enabling advanced services by interconnecting (physical and virtual) things based on existing and evolving interoperable information and communication technologies.” ISO((Thonet et al., 2017),(SWG 5, 2014)) has defined IoT as “An infrastructure of interconnected objects, people, systems and information resources together with intelligent services to allow them to process information of the physical and the virtual world and react.”
These definitions, provided by various authoritative organizations, reflect the diverse applications and the evolving nature of IoT technology. Table 5-2 contrasts the multiple definitions with our definition of IoT system.
Table 5-2: Contrast of Different Definitions of IoT
Organization | Scope and Emphasis | Key Characteristics | Technological Aspects | Service Provision |
IEEE | Self-configuring, adaptive network, standard communication protocols. | Sensing/actuation capabilities, programmability, unique identification. | Standard communication protocols, physical/virtual representation, unique ID. | Services with/without human intervention, unique identification, data capture, communication, and actuation. |
ITU | Global infrastructure, interoperable technologies. | They are interconnecting physical and virtual things. | Existing and evolving interoperable information and communication technologies. | We are enabling advanced services through the interconnection of things. |
ISO | Infrastructure of interconnected objects, people, systems, and information resources. | Interconnected objects, people, systems, information resources, intelligent services. | Intelligent services processing information from physical and virtual worlds. | Intelligent services that process information and react to changes in the physical and virtual worlds. |
Our Definition | Ecosystem integrating systems of systems and networks of networks. | Integration of systems and networks to create intelligent environments. | Combination of systems of systems and networks of networks supporting diverse applications and domains. | Task performance, efficiency improvements, and enhanced decision-making by integrating and connecting various systems and networks. |
Source: Author
Each definition emphasizes different aspects, whether it’s the adaptive and self-configuring capabilities highlighted by IEEE, the global infrastructure and interoperability stressed by ITU, or the intelligent services and interconnected resources underscored by ISO. These perspectives collectively contribute to a comprehensive understanding of IoT’s scope, technological aspects, and significant impact on various representative domains.
At its core, IoT relies on sensors and the ability to make sense of signals /data. Thereby, the core components of IoT are sensors, signal /data processing, communication /connectivity, and cognitive systems.
Sensors are the primary devices that collect data from the physical environment. They can detect and measure various parameters like temperature, humidity, light, motion, and more. Without sensors, IoT devices would be unable to perceive the world around them. They provide the necessary raw data that forms the foundation for all IoT applications.
Effective communication and connectivity are essential for ensuring that data is reliably transmitted across the network, enabling real-time monitoring and control of IoT devices. This component involves the transmission of data collected by sensors to other devices, systems, or central servers. It includes various communication technologies like Wi-Fi, Bluetooth, Zigbee, LoRa, and cellular networks.
Signal and data processing involves transforming raw data from sensors into usable information. This step includes filtering, aggregating, analyzing, and interpreting the data. Data processing is crucial for extracting meaningful insights from raw sensor data, enabling real-time decision-making and automated responses in IoT systems.
Cognitive systems involve advanced algorithms, machine learning, and artificial intelligence that analyze processed data to make intelligent decisions, predictions, and optimizations. These systems add a layer of intelligence to IoT, allowing for predictive maintenance, anomaly detection, personalized user experiences, and more efficient operations.
One way to look at the various core components and the nature of IoT systems is to view them from a layered architecture perspective illustrated in
Figure 5-2. This layered architecture illustrates how the core components of IoT (sensors, communications, signal /data processing, and cognitive systems) are integrated and interact within an IoT system. Each layer represents a specific function that contributes to the overall system’s efficiency and intelligence, highlighting the complex and interconnected nature of IoT.
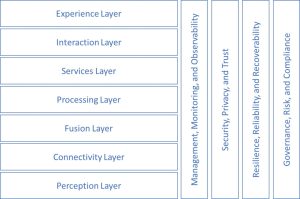
The perception layer is responsible for collecting data from the physical environment. Sensors detect and measure various parameters, while actuators and transducers convert physical quantities into signals and vice versa. By collecting accurate data and enabling real-time control, the perception layer forms the foundation for the entire IoT ecosystem, driving the functionality and efficiency of IoT applications across various domains. This layer’s integration with connectivity, processing, and cognitive systems ensures that IoT systems can provide intelligent, responsive, and automated solutions to real-world challenges.
The connectivity layer ensures reliable data transmission between devices and the central processing units. It involves various communication technologies and network protocols. Advancements across multiple connectivity technologies such as Wi-Fi, Bluetooth, Zigbee, and cellular networks (including 4G and the emergence of 5G) have been crucial. These technologies provide the backbone for device communication in IoT ecosystems, allowing for more reliable and faster data transfer between devices and to the cloud.
The fusion layer processes raw data collected by sensors and 3rd party sources, transforming it into meaningful information for data processing, data modeling, and the use of ontologies. The rise of edge computing, where data processing happens closer to the source of data generation rather than in a centralized cloud, has significantly enhanced IoT capabilities. This approach reduces latency, conserves bandwidth, and improves response times, making IoT systems more efficient and effective, particularly in time-sensitive applications.
The processing layer provides the necessary data processing capabilities to enable analytics and cognitive abilities to further decision-making and trigger some activity.
The services layer provides various services, ensuring access and capabilities across different domains of IoT applications.
The interaction layer facilitates interaction between users and the system through APIs, streaming, messaging protocols, and native interfaces.
The experience layer represents the end-user interface, providing access to IoT functionalities through wearables, web applications, native apps, and intelligent agents.
Cross-Layer Functions
Management and Observability: Ensures the IoT system is manageable and observable, allowing for efficient monitoring and control of various components.
Security, Privacy, and Trust: Maintains the security, privacy, and trustworthiness of the IoT system, safeguarding data and ensuring user trust.
Resilience, Reliability, and Recoverability: Enhances the system’s ability to recover from failures and maintain reliable operations.
Governance, Risk, and Compliance: Ensures that the IoT system adheres to regulatory standards and manages risks effectively.
The layered architecture of IoT systems highlights the inherent complexity of these systems and underscores the need for robust management strategies. Each layer and cross-layer function adds to the overall complexity but also needs mechanisms and technologies to manage and mitigate this complexity. As we navigate the complexities of IoT within the context of humanity, it becomes increasingly vital to comprehend the notion of human-centric technology security.
Understanding Human-Centric Technology Security
A human-centric approach prioritizes the needs, experiences, and values of humans in the development of systems, products, or policies. It suggests that human well-being should be at the forefront of decision-making. It highlights the importance of considering not only the immediate and apparent human needs but also the broader and long-term implications of human-centric designs. It requires an attempt to help humans be aware (contribute to awareness) and understand (contribute to their knowledge) of how the systems work and interact with the environment, making it part of their life and their livelihood. It is this aspect that is a fallacy in our current human-centric designs that mostly measure usability and superficial impacts on the environment. If we need to move towards a world that gels together in harmony, systems need to create an aura of awareness and understanding. The overarching theme is the need for a shift from isolated, narrow-focused designs to holistic solutions that consider the interconnectedness of human activities with the environment. This approach aligns with the idea that accurate human-centric solutions contribute positively not only to individual well-being but also to the collective health of ecosystems.
A holistic approach to human-centric secure IoT systems involves considering the full spectrum of factors that influence the development, deployment, operations /usage, and impact of these technologies. This includes organizational, technical, environmental, social, and economic dimensions that influence the development, deployment, operations, and impact of these technologies. Some considerations may be,
Privacy and Data Protection
Privacy emerges as a central concern in humanitarian technology security, as IoT deployments, particularly at the perception layer, often involve the collection and processing of sensitive personal data. The vulnerabilities in and the proliferation of interconnected devices and sensors increase the risk of data breaches and unauthorized access, underscoring the importance of robust privacy safeguards and data protection mechanisms.
Effective IoT design must incorporate strong data protection measures, such as encryption, secure data storage, and anonymization techniques, to protect against unauthorized access and potential misuse.
Equity and Access
IoT systems, particularly at the interactions and experience layers, can widen the digital divide if specific populations or regions have less access to the benefits these technologies offer. Humanitarian-driven IoT design seeks to ensure equitable access to technology, including affordability, usability for people with disabilities, and availability in diverse linguistic and cultural contexts. This approach helps prevent exacerbating existing inequalities.
Resilience and Reliability
In humanitarian settings, where infrastructure may be fragile and resources limited, ensuring the resilience and reliability of IoT systems is essential. Vulnerabilities in technology infrastructure can have far-reaching consequences, jeopardizing the delivery of crucial services and exacerbating humanitarian crises. Building resilient IoT solutions that can withstand adverse conditions and disruptions is critical for maintaining continuity and effectiveness in humanitarian operations.
Ethical Use and Impact
At the heart of humanitarian technology security lie fundamental ethical and human rights considerations, raising complex questions about consent, autonomy, and accountability. The use of IoT technologies for surveillance, monitoring, and control purposes raises concerns about infringements on individual rights and freedoms, challenging humanitarian principles of impartiality, neutrality, and independence. Designers and deployers need to balance the benefits of IoT solutions against the potential for invasive monitoring or other practices that could infringe on individual freedoms and rights. IEEE Ethically Assisted Design can help in this context.
Environmental Impact
IoT systems contribute to electronic waste and can have significant environmental impacts throughout their lifecycle, from manufacturing to disposal. Humanitarian-focused IoT design emphasizes sustainability, including using environmentally friendly materials, designing for energy efficiency, and facilitating easy recycling of devices.
Collaborative Governance and Accountability
Effective governance mechanisms are vital for ensuring accountability and transparency in humanitarian technology deployments. Collaborative approaches that involve all stakeholders, including affected communities, in decision-making processes can help foster trust and mitigate risks. Additionally, mechanisms for monitoring and accountability are necessary to address potential misuse or abuse of technology and ensure that humanitarian principles are upheld.
Understanding humanitarian technology security within the IoT landscape requires a holistic approach that considers ethical, societal, and humanitarian implications. By navigating the complex interplay of security concerns and human values, we can work towards harnessing the potential of IoT to advance human-centric objectives while safeguarding the rights and dignity of individuals and communities. Doing so not only mitigates potential harms but also maximizes the positive impacts of these technologies on society.
Stakeholder Engagement and Community Involvement
Effective stakeholder engagement and community involvement are essential for the success of human-centric IoT systems. Engaging with local communities, humanitarian organizations, government agencies, and other relevant stakeholders allows for a more holistic understanding of the challenges and opportunities faced by vulnerable populations. By actively involving end-users in the design, testing, and implementation of IoT solutions, organizations can ensure that their initiatives are contextually relevant, culturally appropriate, and sustainable in the long term. Furthermore, engaging with communities fosters trust, ownership, and accountability, laying the foundation for collaborative partnerships that drive positive change.
By embracing strategies that prioritize humanitarian considerations, stakeholder engagement, and inclusivity, organizations can foster a more humanitarian-centric approach to IoT that maximizes social impact and promotes sustainable development. By integrating these principles into the design, development, and implementation of IoT solutions, stakeholders can empower communities, address pressing humanitarian challenges, and build a more equitable and resilient future for all.
SUEZ CANAL INCIDENT
On 23 March 2021, the Suez Canal, one of the world’s busiest maritime trade routes, was blocked by the container ship Ever Given (Chellel et al., 2021). The vessel ran aground and became lodged across the canal, disrupting global trade for six days. This incident had significant technical, environmental, social, and economic implications.
The Ever Given(Vessel Characteristics, n.d.), one of the largest container ships in the world, became lodged sideways in the canal due to strong winds and poor visibility, blocking one of the busiest maritime trade routes for almost a week. It led to a significant disruption in global trade, as hundreds of ships were delayed or had to reroute around the Cape of Good Hope. It took several days and a coordinated effort involving tugboats, dredgers, and excavators to free the vessel and reopen the canal to traffic. This incident underscored the complex interplay between human factors, technological dependencies, and the humanitarian implications of maritime accidents(Lee & Wong, 2021; Lutmar & Rubinovitz, 2023; Panama Maritime Authority, 2023).
Ever Given’s investigative report highlights the complex situation the crew and various stakeholders in maneuvering the ship through the Suez Canal found themselves in. It highlights environmental factors, potential systemic failures, and possible human barriers like language difficulties. The report also highlights other vital factors in critical infrastructure operations, including the spread of key stakeholders across the globe. The report concludes by identifying the existential risk of “vessel grounding” during transiting canals and a potential safety awareness review regarding navigations in the canal. Investigative reporting from the NYTimes(Yee & Glanz, 2021) highlights some shortcomings in finding solutions to mitigate such incidents due to the complexity and complications of commercial maritime business and operations.
Hidden in the references is the crucial role automatic identification systems[3] (AIS)(Shipboard Automatic Identification System Displays, 2003) with the help of satellite and ship communications played a role in assisting safety investigators in reconstructing the incident, helping investigators understand the sequence of events and identify key contributing factors to the incident. The analysis highlighted several shortcomings in current operations, including decision-making under extreme weather conditions, communication issues, and the need for better integration of environmental data.
Reconstruction of Events
- Data Utilization: AIS data was used to track the vessel’s movements and identify the exact moment it ran aground.
- Shortcomings Identified: Decision-making processes under extreme weather conditions were scrutinized, highlighting the need for better support systems.
Communication Issues
- Data Insights: Analysis of AIS data revealed communication breakdowns between the crew and pilots.
- Improvements Suggested: Enhanced communication protocols and real-time data sharing could prevent such issues.
Environmental Data Integration
- Findings: AIS data combined with weather reports showed how environmental conditions contributed to the incident.
- Recommendations: Integration of real-time environmental data into AIS to provide comprehensive situational awareness.
To enable a better human-centric secure system, we may require multiple approaches to implement future enhancements to existing systems. When considering purely technical considerations, a holistic approach to prioritizing and implementing improvements can require the use of more than one method (see Table 5-3) based on their distinct strengths in addressing different aspects of significant, complex, distributed system failures. Our rationale in choosing these methods was based on a need to address safety, dynamic interactions, scenario outcomes, and spec-multifaceted comprehensively. Such a multifaceted approach also enhances understanding and helps develop robust end-to-end solutions to prevent similar incidents in the future.
Table 5-3: Use Of Multiple Methods To Arrive At Holistic Approach
Method | Purpose | Strengths | Application |
STPA (Systems-Theoretic Process Analysis)(Leveson, 2011) | Ensures system safety by identifying and mitigating unsafe actions | · Safety-Focused: Identifies unsafe control actions and helps design safety constraints.
· System-Level View: Analyzes interactions within the entire control structure. |
Suitable for identifying and mitigating safety hazards in AIS operations and decision-making processes.
· Identify Unsafe Control Actions: Analyze the AIS-related control actions during the incident, such as miscommunication and inadequate response to environmental data. · Design Safety Constraints: Develop constraints like mandatory real-time updates and enhanced pilot training. Example: Highlight how better integration of AIS with environmental sensors could provide more accurate data to pilots, reducing the chance of navigational errors. |
CLD (Causal Loop Diagrams)(Barbrook-Johnson & Penn, 2022) | Provides a holistic view of dynamic interactions and feedback loops | · Feedback and Dynamics: Visualizes cause-and-effect relationships and feedback loops.
· System Behavior: Helps understand how different variables interact over time. |
Useful for illustrating the dynamic interactions between AIS data, environmental conditions, and decisions.
· Visualize Interactions: Create diagrams to map out the interaction between AIS data, pilot decisions, and environmental conditions. · Identify Feedback Loops: Show how increased speed in response to high winds could lead to further instability. Example: Illustrate a feedback loop where better AIS data improves pilot decision-making, reducing the likelihood of incidents. |
ETA (Event Tree Analysis)[4] | Analyzes potential scenarios and their probabilities | · Scenario Analysis: Explores different outcomes from a single initiating event.
· Probability Assessment: Evaluates the likelihood of various outcomes. |
· Effective in assessing the potential outcomes of pilot decisions under extreme weather conditions.
· Evaluate the role of AIS data in decision-making processes.
· Analyze Outcomes: Assess the different outcomes of pilot decisions under extreme weather conditions using AIS data. · Evaluate Mitigation Measures: Consider how enhanced AIS capabilities could change the event tree to prevent grounding. Example: Map the event tree from the decision to increase speed, showing how improved data and decision-support tools could lead to safer outcomes. |
FMEA (Failure Mode and Effects Analysis) | Identifies and prioritizes specific failures and mitigations | · Detailed Failure Analysis: Identifies all potential failure modes and their effects.
· Prioritization: Ranks failures by severity, occurrence, and detectability. |
It helps identify specific AIS failures, such as communication breakdowns, and prioritize mitigation strategies.
· Identify Failure Modes: Examine potential AIS failures, such as delays in data transmission or inaccuracies in environmental data integration. · Prioritize Mitigations: Rank these failures and develop targeted improvements. Example: Identify a failure mode where outdated AIS data contributes to poor decision-making and propose regular updates and system checks. |
Source: Author
Application of STPA (Systems-Theoretic Process-Analysis) to Improve Decision-Making Under Extreme Weather Conditions
Goal: Enhance decision-making processes and support systems for maritime navigation during extreme weather conditions using STPA.
Step 1: Define the Purpose and Scope
System: Maritime navigation system, including AIS, environmental sensors, and communication protocols.
Purpose: Ensure safe navigation and effective decision-making during extreme weather conditions.
Scope: Focus on interactions between AIS, crew, pilots, and environmental data.
Step 2: Identify Potential Hazards
Hazards:
- H-1: Navigational errors due to misinterpretation of AIS data during extreme weather.
- H-2: Delayed response to changing environmental conditions.
- H-3: Miscommunication between crew and pilots leading to incorrect maneuvers.
Step 3: Identify Unsafe Control Actions (UCAs)
UCAs:
- UCA-1: Pilots increase speed without considering updated environmental data.
- UCA-2: Crew fails to relay critical environmental updates to pilots.
- UCA-3: AIS data not adequately integrated with real-time weather information.
Step 4: Develop a Control Structure
Control Structure Components:
- AIS and Environmental Sensors: Provide real-time data on ship position and environmental conditions.
- Decision-Support Tools: Analyze data and offer recommendations.
- Communication Systems: Ensure clear and continuous information flow between crew and pilots.
- Training Programs: Enhance crew and pilot understanding of system interactions and decision-making.
Step 5: Analyze the Control Structure for Unsafe Control Actions
Analysis:
- AIS and Environmental Sensors:
- Potential Failure: Inaccurate or outdated data leading to poor decision-making.
- Control Action: Implement regular updates and maintenance.
- Decision-Support Tools:
- Potential Failure: Tools fail to provide timely or accurate recommendations.
- Control Action: Integrate machine learning algorithms to improve predictive capabilities.
- Communication Systems
- Potential Failure: Miscommunication or delayed information sharing.
- Control Action: Establish redundant communication channels and protocols.
- Training Programs
- Potential Failure: Inadequate training on using decision-support tools.
- Control Action: Develop comprehensive training modules and simulation exercises.
Step 6: Identify and Implement Safety Constraints
Safety Constraints:
- SC-1: Ensure AIS and environmental sensors provide real-time, accurate data.
- SC-2: Decision-support tools must be updated regularly to reflect the latest data.
- SC-3: Communication protocols must be clear and followed strictly.
- SC-4: Regular training sessions must be conducted for all crew members and pilots.
By applying STPA, we can identify unsafe control actions and design safety constraints to improve decision-making processes during extreme weather conditions. Enhancing the integration of real-time data, decision-support tools, communication systems, and training programs will help mitigate the risks identified and ensure safer maritime navigation.
HL Architectural Enhancements
For the technology architecture, a few components may need to be enhanced to facilitate better navigation under extreme weather conditions.
Perception Layer (with Security and Observability considerations)
- Environmental Sensors: Accurate real-time data on weather conditions (wind speed, visibility, tides).
- Navigational Sensors: Real-time data on ship position, speed, and course.
Network Layer (with Security and Resilience considerations)
- Reliable Communication Channels: Ensure continuous data transmission between shipboard systems, crew, pilots, and shore-based control centers.
- Redundant Communication Systems: Backup channels to prevent data loss during communication failures.
Fusion Layer (with Governance, Risk and Compliance considerations)
- Edge Computing: Process data closer to the source for real-time analysis and reduced latency.
- Machine Learning: Predictive analytics for decision support and hazard prediction.
Processing Layer (with Governance, Risk, and Compliance considerations)
- Decision-Support Tools: Real-time analytics and recommendations based on integrated AIS and environmental data.
- Management Systems: Coordination of operations, maintenance schedules, and response protocols.
Services Layer (with Security and Governance considerations)
- Training Simulators: Simulation-based training programs to enhance decision-making skills under extreme weather conditions.
Experience Layer (with Security considerations)
- User-Friendly Interfaces: Intuitive interfaces reduce cognitive load on the crew, especially during high-stress situations.
Interaction Layer
- Orchestration and choreography for culture and language adaptations
Governance, Risk, and Compliance
- Compliance and Safety Standards: Ensure adherence to maritime safety regulations and continuous improvement based on incident analysis.
- Language Barriers
Security, Privacy, and Trust
- Data Security: Protect data integrity
- Access Control: Ensure only authorized personnel can access critical data and control systems.
Summary
This chapter has provided a short exploration of the intersection between IoT technology and humanitarian values, emphasizing the importance of balancing technological advancement with human-centric considerations. The intersection of IoT and humanitarianism presents a vast array of opportunities for collaboration, innovation, and impact. By fostering interdisciplinary partnerships, investing in research and development, and embracing a human-centric approach to technology, we can build a more inclusive, resilient, and equitable future for all.
In closing, let us remember that the accurate measure of our success lies not only in technological advancement but in our ability to harness the power of IoT for the greater good. By staying true to our humanitarian values and forging ahead with responsibility and foresight, we can pave the way for a brighter tomorrow—one where technology serves humanity in meaningful and transformative ways.
References
Abbasi, M., Yaghmaee, M. H., & Rahnama, F. (2019). Internet of Things in agriculture: A survey. 2019 3rd International Conference on Internet of Things and Applications (IoT), 1–12. https://doi.org/10.1109/IICITA.2019.8808839
Abegaz, B. W., Datta, T., & Mahajan, S. M. (2018). Sensor technologies for the energy-water nexus – A review. Applied Energy, 210, 451–466. https://doi.org/10.1016/j.apenergy.2017.01.033
Abir, S. M. A. A., Anwar, A., Choi, J., & Kayes, A. S. M. (2021). IoT-Enabled Smart Energy Grid: Applications and Challenges. IEEE Access, 9, 50961–50981. https://doi.org/10.1109/ACCESS.2021.3067331
Alavi, A. H., Jiao, P., Buttlar, W. G., & Lajnef, N. (2018). Internet of Things-enabled smart cities: State-of-the-art and future trends. Measurement, 129, 589–606. https://doi.org/10.1016/j.measurement.2018.07.067
Almalki, Faris. A., Alsamhi, S. H., Sahal, R., Hassan, J., Hawbani, A., Rajput, N. S., Saif, A., Morgan, J., & Breslin, J. (2023). Green IoT for Eco-Friendly and Sustainable Smart Cities: Future Directions and Opportunities. Mobile Networks and Applications, 28(1), 178–202. https://doi.org/10.1007/s11036-021-01790-w
Al-rawashdeh, M., Keikhosrokiani, P., Belaton, B., Alawida, M., & Zwiri, A. (2022). IoT Adoption and Application for Smart Healthcare: A Systematic Review. Sensors (Basel, Switzerland), 22(14), 5377. https://doi.org/10.3390/s22145377
Anagnostopoulos, T., Zaslavsky, A., Kolomvatsos, K., Medvedev, A., Amirian, P., Morley, J., & Hadjieftymiades, S. (2017). Challenges and Opportunities of Waste Management in IoT-Enabled Smart Cities: A Survey. IEEE Transactions on Sustainable Computing, 2(3), 275–289. https://doi.org/10.1109/TSUSC.2017.2691049
Atzori, L., Iera, A., & Morabito, G. (2010). The Internet of Things: A survey. Computer Networks, 54(15), 2787–2805. https://doi.org/10.1016/j.comnet.2010.05.010
Atzori, L., Iera, A., & Morabito, G. (2017). Understanding the Internet of Things: Definition, potentials, and societal role of a fast-evolving paradigm. Ad Hoc Networks, 56, 122–140. https://doi.org/10.1016/j.adhoc.2016.12.004
Babun, L., Denney, K., Celik, Z. B., McDaniel, P., & Uluagac, A. S. (2021). A survey on IoT platforms: Communication, security, and privacy perspectives. Computer Networks, 192, 108040. https://doi.org/10.1016/j.comnet.2021.108040
Baker, S. B., Xiang, W., & Atkinson, I. (2017). Internet of Things for Smart Healthcare: Technologies, Challenges, and Opportunities. IEEE Access, 5, 26521–26544. https://doi.org/10.1109/ACCESS.2017.2775180
Barbrook-Johnson, P., & Penn, A. S. (2022). Causal Loop Diagrams. In P. Barbrook-Johnson & A. S. Penn (Eds.), Systems Mapping: How to build and use causal models of systems (pp. 47–59). Springer International Publishing. https://doi.org/10.1007/978-3-031-01919-7_4
Bellavista, P., Cardone, G., Corradi, A., & Foschini, L. (2013). Convergence of MANET and WSN in IoT Urban Scenarios. IEEE Sensors Journal, 13(10), 3558–3567. https://doi.org/10.1109/JSEN.2013.2272099
Chellel, K., Campbell, M., & Oanh Ha, K. (2021, 24 June). Six Days in Suez: The Inside Story of the Ship That Broke Global Trade. Bloomberg.Com. https://www.bloomberg.com/news/features/2021-06-24/how-the-billion-dollar-ever-given-cargo-ship-got-stuck-in-the-suez-canal
Čolaković, A., & Hadžialić, M. (2018). Internet of Things (IoT): A review of enabling technologies, challenges, and open research issues. Computer Networks, 144, 17–39. https://doi.org/10.1016/j.comnet.2018.07.017
De Sanctis, M., Cianca, E., Araniti, G., Bisio, I., & Prasad, R. (2016). Satellite Communications Supporting Internet of Remote Things. IEEE Internet of Things Journal, 3(1), 113–123. https://doi.org/10.1109/JIoT.2015.2487046
Derawi, M., Dalveren, Y., & Cheikh, F. A. (2020). Internet-of-Things-Based Smart Transportation Systems for Safer Roads. 2020 IEEE 6th World Forum on Internet of Things (WF-IoT), 1–4. https://doi.org/10.1109/WF-IoT48130.2020.9221208
Farahani, B., Firouzi, F., Chang, V., Badaroglu, M., Constant, N., & Mankodiya, K. (2018). Towards fog-driven IoT eHealth: Promises and challenges of IoT in medicine and healthcare. Future Generation Computer Systems, 78, 659–676. https://doi.org/10.1016/j.future.2017.04.036
Feki, M. A., Kawsar, F., Boussard, M., & Trappeniers, L. (2013). The Internet of Things: The Next Technological Revolution. Computer, 46(2), 24–25. https://doi.org/10.1109/MC.2013.63
Friha, O., Ferrag, M. A., Shu, L., Maglaras, L., & Wang, X. (2021). Internet of Things for the Future of Smart Agriculture: A Comprehensive Survey of Emerging Technologies. IEEE/CAA Journal of Automatica Sinica, 8(4), 718–752. https://doi.org/10.1109/JAS.2021.1003925
Gao, J., Li, D., & Havlin, S. (2014). From a single network to a network of networks. National Science Review, 1(3), 346–356. https://doi.org/10.1093/nsr/nwu020
Gerla, M., Lee, E.-K., Pau, G., & Lee, U. (2014). Internet of vehicles: From intelligent grid to autonomous cars and vehicular clouds. 2014 IEEE World Forum on Internet of Things (WF-IoT), 241–246. https://doi.org/10.1109/WF-IoT.2014.6803166
Javaid, M., & Khan, I. H. (2021). Internet of Things (IoT) enabled healthcare to help take on the challenges of the COVID-19 pandemic. Journal of Oral Biology and Craniofacial Research, 11(2), 209–214. https://doi.org/10.1016/j.jobcr.2021.01.015
Kelly, J. T., Campbell, K. L., Gong, E., & Scuffham, P. (2020). The Internet of Things: Impact and Implications for Health Care Delivery. Journal of Medical Internet Research, 22(11), e20135. https://doi.org/10.2196/20135
Khanboubi, F., Boulmakoul, A., & Tabaa, M. (2019). Impact of digital trends using IoT on banking processes. Procedia Computer Science, 151, 77–84. https://doi.org/10.1016/j.procs.2019.04.014
Kirmani, S., Mazid, A., Khan, I. A., & Abid, M. (2023). A Survey on IoT-Enabled Smart Grids: Technologies, Architectures, Applications, and Challenges. Sustainability, 15(1), Article 1. https://doi.org/10.3390/su15010717
Kramp, T., van Kranenburg, R., & Lange, S. (2013). Introduction to the Internet of Things. In A. Bassi, M. Bauer, M. Fiedler, T. Kramp, R. van Kranenburg, S. Lange, & S. Meissner (Eds.), Enabling Things to Talk: Designing IoT solutions with the IoT Architectural Reference Model (pp. 1–10). Springer. https://doi.org/10.1007/978-3-642-40403-0_1
Krotov, V. (2017). The Internet of Things and new business opportunities. Business Horizons, 60(6), 831–841. https://doi.org/10.1016/j.bushor.2017.07.009
Kua, J., Loke, S. W., Arora, C., Fernando, N., & Ranaweera, C. (2021). Internet of Things in Space: A Review of Opportunities and Challenges from Satellite-Aided Computing to Digitally Enhanced Space Living. Sensors (Basel, Switzerland), 21(23), 8117. https://doi.org/10.3390/s21238117
Kusiak, A. (2018). Smart manufacturing. International Journal of Production Research, 56(1–2), 508–517. https://doi.org/10.1080/00207543.2017.1351644
Lande, R. S., Meshram, S. A., & Deshmukh, P. P. (2018). Smart banking using IoT. 2018 International Conference on Research in Intelligent and Computing in Engineering (RICE), 1–4. https://doi.org/10.1109/RICE.2018.8627903
Lee, J. M., & Wong, E. Y. (2021). Suez Canal blockage: An analysis of legal impact, risks and liabilities to the global supply chain. MATEC Web of Conferences, 339, 01019. https://doi.org/10.1051/matecconf/202133901019
Leveson, N. (2011). Engineering a safer world: Systems thinking applied to safety. MIT Press.
Li, B., Chen, R., & Wang, H. C. (2021). Using intelligent prediction machine and dynamic workflow for banking customer satisfaction in IoT environment. Journal of Ambient Intelligence and Humanized Computing. https://doi.org/10.1007/s12652-021-03201-0
Li, S., Xu, L. D., & Zhao, S. (2015). The Internet of Things: A survey. Information Systems Frontiers, 17(2), 243–259. https://doi.org/10.1007/s10796-014-9492-7
Lin, J., Yu, W., Zhang, N., Yang, X., Zhang, H., & Zhao, W. (2017). A Survey on Internet of Things: Architecture, Enabling Technologies, Security and Privacy, and Applications. IEEE Internet of Things Journal, 4(5), 1125–1142. https://doi.org/10.1109/JIoT.2017.2683200
Lutmar, C., & Rubinovitz, Z. (Eds.). (2023). The Suez Canal: Past Lessons and Future Challenges. Springer International Publishing. https://doi.org/10.1007/978-3-031-15670-0
Maier, M. W. (1996). Architecting Principles for Systems‐of‐Systems. INCOSE International Symposium, 6(1), 565–573. https://doi.org/10.1002/j.2334-5837.1996.tb02054.x
Minerva, R., Biru, A., & Rotondi, D. (2015). Towards a Definition of the Internet of Things. IEEE Internet Initiative. https://iot.ieee.org/definition.html
Minoli, D., Sohraby, K., & Occhiogrosso, B. (2017). IoT Considerations, Requirements, and Architectures for Smart Buildings—Energy Optimization and Next-Generation Building Management Systems. IEEE Internet of Things Journal, 4(1), 269–283. https://doi.org/10.1109/JIoT.2017.2647881
Miraz, M. H., Ali, M., Excell, P. S., & Picking, R. (2015). A review of the Internet of Things (IoT), Internet of Everything (IoE), and Internet of Nano Things (IoT). 2015 Internet Technologies and Applications (ITA), 219–224. https://doi.org/10.1109/ITechA.2015.7317398
Panama Maritime Authority. (2023). Maritime Safety Investigative Report: Grounding of MV Ever Given At Suez Canal Egypt on 23 March 2021 (Investigative M/V “Ever Given” R-026-2021-DIAM; p. 68). Maritime Affairs Investigative Department, General Directorate of Merchant Marine, Panama Maritime Authority. https://drive.google.com/file/d/1P-Qk3DzPCDBaSMWi7qBjSaWaYrwVXb2D/view
Pramudhita, A. N., Asmara, R. A., Siradjuddin, I., & Rohadi, E. (2018). Internet of Things Integration in Smart Grid. 2018 International Conference on Applied Science and Technology (iCAST), 718–722. https://doi.org/10.1109/iCAST1.2018.8751518
Putra, M. P. (2021). An Analysis of Big Data Analytics, IoT and Augmented Banking on Consumer Loan Banking Business in Germany. Journal of Research on Business and Tourism, 1(1), Article 1. https://doi.org/10.37535/104001120212
Qu, Z., Zhang, G., Cao, H., & Xie, J. (2017). LEO Satellite Constellation for Internet of Things. IEEE Access, 5, 18391–18401. https://doi.org/10.1109/ACCESS.2017.2735988
Rajak, P., Ganguly, A., Adhikary, S., & Bhattacharya, S. (2023). Internet of Things and smart sensors in agriculture: Scopes and challenges. Journal of Agriculture and Food Research, 14, 100776. https://doi.org/10.1016/j.jafr.2023.100776
Ray, P. P. (2022). A review on 6G for space-air-ground integrated network: Key enablers, open challenges, and future direction. Journal of King Saud University – Computer and Information Sciences, 34(9), 6949–6976. https://doi.org/10.1016/j.jksuci.2021.08.014
Rejeb, A., Rejeb, K., Simske, S., Treiblmaier, H., & Zailani, S. (2022). The big picture on the Internet of Things and the smart city: A review of what we know and what we need to know. Internet of Things, 19, 100565. https://doi.org/10.1016/j.iot.2022.100565
Ren, C. H. (2017). How Systems Form and How Systems Break (Vol. 72). Springer International Publishing. https://doi.org/10.1007/978-3-319-44030-9
Sanchez, L., Muñoz, L., Galache, J. A., Sotres, P., Santana, J. R., Gutierrez, V., Ramdhany, R., Gluhak, A., Krco, S., Theodoridis, E., & Pfisterer, D. (2014). SmartSantander: IoT experimentation over a smart city testbed. Computer Networks, 61, 217–238. https://doi.org/10.1016/j.bjp.2013.12.020
Shih, C.-S., Chou, J.-J., Reijers, N., & Kuo, T.-W. (2016). Designing CPS/IoT applications for smart buildings and cities. IET Cyber-Physical Systems: Theory & Applications, 1(1), 3–12. https://doi.org/10.1049/iet-cps.2016.0025
Shipboard Automatic Identification System Displays: Meeting the Needs of Mariners — Special Report 273. (2003). Transportation Research Board. https://doi.org/10.17226/10708
Singh, A. K. (2022). Smart Farming: Applications of IoT in Agriculture. In C. M. Hussain & P. Di Sia (Eds.), Handbook of Smart Materials, Technologies, and Devices: Applications of Industry 4.0 (pp. 1655–1687). Springer International Publishing. https://doi.org/10.1007/978-3-030-84205-5_114
Singh, N., & Singh, A. N. (2020). Odysseys of agriculture sensors: Current challenges and forthcoming prospects. Computers and Electronics in Agriculture, 171, 105328. https://doi.org/10.1016/j.compag.2020.105328
Singhvi, R. K., Lohar, R. L., Kumar, A., Sharma, R., Sharma, L. D., & Saraswat, R. K. (2019). IoT-based innovative Waste Management System: India perspective. 2019 4th International Conference on Internet of Things: Smart Innovation and Usages (IoT-SIU), 1–6. https://doi.org/10.1109/IoT-SIU.2019.8777698
Sobin, C. C. (2020). A Survey on Architecture, Protocols, and Challenges in IoT. Wireless Personal Communications, 112(3), 1383–1429. https://doi.org/10.1007/s11277-020-07108-5
Soori, M., Arezoo, B., & Dastres, R. (2023). Internet of things for smart factories in industry 4.0, a review. Internet of Things and Cyber-Physical Systems, 3, 192–204. https://doi.org/10.1016/j.iotcps.2023.04.006
SWG 5, A. (2014). Internet of Things. ISO /IEC JTC 1.
Tao, F., Qi, Q., Liu, A., & Kusiak, A. (2018). Data-driven smart manufacturing. Journal of Manufacturing Systems, 48, 157–169. https://doi.org/10.1016/j.jmsy.2018.01.006
Thonet, G., Information, H. of, Standards, C. T., & IEC. (2017, 15 July). Driving the Internet of Things through standardization. IEC E-Tech. https://etech.iec.ch/issue/2017-07/driving-the-internet-of-things-through-standardization
Ushakov, D., Dudukalov, E., Kozlova, E., & Shatila, K. (2022). The impact of the Internet of Things on smart public transportation. Transportation Research Procedia, 63, 2392–2400. https://doi.org/10.1016/j.trpro.2022.06.275
Vessel Characteristics: Ship EVER GIVEN (Container Ship) Registered in Panama – Vessel details, Current position, and Voyage information – IMO 9811000MMSI 9811000Call Sign H3RC | AIS Marine Traffic. (n.d.). MarineTraffic.Com. Retrieved 5 June 2024, from https://www.marinetraffic.com/en/ais/details/ships/shipid:5630138/mmsi:353136000/imo:9811000/vessel:EVER GIVEN
Y.2060: Overview of the Internet of Things. (n.d.). Retrieved 24 June 2024, from https://www.itu.int/rec/T-REC-Y.2060-201206-I
Yang, H., Kumara, S., Bukkapatnam, S. T. S., & Tsung, F. (2019). The Internet of Things for Smart Manufacturing: A review. IISE Transactions, 51(11), 1190–1216. https://doi.org/10.1080/24725854.2018.1555383
Yee, V., & Glanz, J. (2021, 17 July). How One of the World’s Biggest Ships Jammed the Suez Canal. The New York Times. https://www.nytimes.com/2021/07/17/world/middleeast/suez-canal-stuck-ship-ever-given.html
Zanella, A., Bui, N., Castellani, A., Vangelista, L., & Zorzi, M. (2014). Internet of Things for Smart Cities. IEEE Internet of Things Journal, 1(1), 22–32. https://doi.org/10.1109/JIoT.2014.2306328
Zantalis, F., Koulouras, G., Karabetsos, S., & Kandris, D. (2019). A Review of Machine Learning and IoT in Smart Transportation. Future Internet, 11(4), Article 4. https://doi.org/10.3390/fi11040094
End Notes
[1] During John B. Kennedy’s interview on 30 January 1926, Tesla describes his vision of the vest-pocket equipment, https://www.tfcbooks.com/tesla/1926-01-30.htm
[2] Also, see their website, https://iot.ieee.org/definition.html
[3] The use of which can be viewed on the site, https://www.marinetraffic.com/en/ais/home/centerx:-12.0/centery:25.0/zoom:4
[4] Any good book on reliability gives information on event trees and FMEA. For example, https://handbook.reliability.space/en/latest/home.html or https://ntrs.nasa.gov/api/citations/20150022337/downloads/20150022337.pdf