2 Mobility (Murthy)
Introduction
In today’s interconnected world, telecommunication technologies have evolved staggeringly, reshaping how individuals, businesses, and societies communicate, collaborate, and function globally. From broadband internet to cellular networks, wireless technologies, and satellite systems, these advancements have ushered in an era of unprecedented connectivity and information exchange, fundamentally altering how humans live and work. Mobility, once synonymous with physical movement, now encompasses digital connectivity and accessibility, facilitated by interconnected technologies enabling seamless communication, information access, and empowerment across global populations.
Amidst this rapid technological evolution, a pressing need arises to critically examine the intersection of mobility, humanity, and human-centricity. Humanity, defined by our collective values and aspirations, must guide the development and deployment of inherently human-centric technologies – designed not only for efficiency but also to enrich human experiences and uphold ethical standards.
Consider the application of AirTags in modern travel scenarios, where these small, location-tracking devices revolutionize how we manage luggage. By attaching AirTags to suitcases and travel bags, travelers gain peace of mind knowing they can quickly locate their belongings if misplaced or lost. This innovation exemplifies human centricity, offering a practical solution that enhances convenience and reduces stress during travel, thereby improving the overall travel experience.
In parallel, while AirTags uses Bluetooth for nearby device connectivity, the broader applications of GPS technology extend beyond personal convenience. GPS-enabled devices, unlike AirTags, directly harness the satellite network for precise location tracking, supporting everything from navigation to asset management across industries. Companies leverage GPS to monitor the movement of valuable assets like shipping containers and vehicles in real-time, enhancing operational efficiency and minimizing losses due to theft or misplacement. This dual approach showcases how technology tailored to meet specific human needs and business objectives can lead to profound advancements in mobility and security.
In this context, human-centricity involves designing and implementing technologies that resonate with human values, promote inclusivity, and address ethical considerations. It encourages the development of solutions that empower individuals, enhance safety, and contribute to sustainable practices. By exploring how these technologies can facilitate connectivity, efficiency, and accountability, we embark on a journey to harness innovation for the collective benefit of humanity.
This chapter aims to delve deeply into the mechanisms, applications, benefits, and ethical considerations surrounding mobility technologies, using location-based services such as AirTags and GPS tracking as illustrative examples. It seeks insights into their pivotal role in shaping a more connected, equitable, and resilient global society where technological progress harmoniously with human values and aspirations.
Understanding Mobility
Mobility is fundamental to human existence, enabling individuals to access opportunities, resources, and experiences across geographic locations (see, for example, (Alessandretti et al., 2020; Barbosa et al., 2018)). At its core, mobility is the ability to move freely and efficiently, encompassing physical and virtual movements. While traditionally associated with transportation systems and infrastructure, mobility has expanded in the digital age to include connectivity and access to information through mobile technologies.
Understanding mobility encompasses a broad and interdisciplinary exploration of how individuals, goods, and information move between physical and digital spaces. Physical spaces facilitate the movement of people, goods, and services using various modes of transportation. This physical movement can be tracked using geographical coordinates from geographical information systems within the digital spaces. Digital spaces encompass the interactions and activities in virtual environments facilitated by telecommunication and other technologies. Digital spaces provide the ability to access information, services, and resources through enabling digital technologies.
Digital technologies encompass various innovations, including telecommunication and internet technologies. The journey of telecommunication began with the invention of the telegraph in the 19th century, heralding the era of long-distance communication. Innovations such as the telephone, radio, and television paved the way for the digital revolution that unfolded in the late 20th century.
Wireless communications, which involve sending signals over radio waves, a part of the electromagnetic spectrum, and optical communications, utilizing light waveforms within the electromagnetic spectrum, have been pivotal in the proliferation of telecommunication innovations since the 1990s, achieving widespread adoption globally. The basis for wireless communications is rooted in Maxwell’s equations(Griffiths, 2017), which are a set of fundamental equations that describe how electric charges (like the ones in batteries or electrical wires) and magnetic fields (like the ones in magnets) interact and move through space. This forms the root of cellular, Wi-Fi, personal, and satellite communications relying on these electromagnetic waves to transmit signals over the air without physical connections, enabling global connectivity and digital spaces.
Wireless communications use the spectrum, allowing electromagnetic waves to carry signals between devices without physical wires. All wireless devices, such as smartphones, routers, satellites, and others, transmit and receive signals within these allocated frequency bands. Governments regulate spectrum allocation and usage through their regulatory agencies (e.g., FCC[1] in the USA, ISED /CRTC[2] in Canada, OfCom[3] in the UK, and Telecom Regulatory Authority of India[4]). ITU Radiocommunications Sector (ITU-R)[5] globally manages the radio-frequency spectrum and satellite orbit resources. Spectrum enables global connectivity.
Different parts of the electromagnetic spectrum are allocated into specific radio frequency bands(Miao et al., 2016). These bands are used for various wireless communication technologies, such as:
Cellular Networks: Use bands like 900 MHz, 2.3 GHz, 3.5 GHz, 5.2 GHz, and other frequencies
Wi-Fi: frequently accessed using IEEE 802.11(IEEE802, n.d.) protocols, operates in unlicensed bands like 860 MHz, 2.4 GHz, 3.65 GHz, and 5 GHz.
Bluetooth: Uses 2.4 GHz band(Bluetooth Technology Website | The Official Website of Bluetooth Technology., n.d.)
Satellite Communication: Uses different bands(State of the Art of Small Spacecraft Technology, n.d.) for uplink and downlink communications, including C-band, Ku-band, and Ka-band.
Broadcasting: Uses specific bands for TV and radio broadcasting[6]
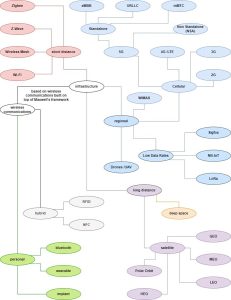
Figure 2-1 provides a comprehensive overview of the various types of wireless communication systems, categorized based on different aspects of Maxwell’s framework for electromagnetic fields and waves. It starts from personal, short-distance communications and progresses to more complex and extensive networks, including deep space and satellite communications. It is also an attempt to showcase the breadth and depth of wireless communication systems and potentially serve as a valuable reference for understanding how various wireless technologies fit into the larger framework of electromagnetic principles. This structure enhances the understanding of the different technologies, their roles, and their impact on humanity and human-centric applications. By accurately positioning each technology, the diagram is a valuable reference for studying and leveraging wireless communication systems to analyze or simulate potential impacts on humanity and human-centricity. The proceeding tabulations briefly describe the technology, a single-liner bullet on its impact on humankind and human-centricity. The reader can crawl the many standard bodies, journal publications, and other sources to gain insights into these vast wireless communication areas.
Table 2-1_Personal Wireless Communications
Technology | Description | Impact | Human-Centricity |
---|---|---|---|
Bluetooth | A short-range wireless technology used for exchanging data between fixed and mobile devices | Enhances connectivity between personal devices like headphones, fitness trackers, and smart home devices | Improves quality of life by enabling wireless connections and data sharing |
Wearables | Devices that can be worn on the body, using wireless communication for health monitoring, fitness tracking, etc., can be part of Body Area Networks[7] | Provides health monitoring, fitness tracking, and real-time data analytics | Empowers individuals to manage their health proactively |
Implant | Usually, a medical device implanted in the body uses wireless communication to transmit data to external receivers. This may be part of Body Area Network (BAN) | Enables advanced medical monitoring and treatment | Enhances patient care through continuous monitoring |
Table 2-1: Personal Wireless Communications
Technology | Description | Impact | Human-Centricity |
---|---|---|---|
Bluetooth | A short-range wireless technology used for exchanging data between fixed and mobile devices | Enhances connectivity between personal devices like headphones, fitness trackers, and smart home devices | Improves quality of life by enabling wireless connections and data sharing |
Wearables | Devices that can be worn on the body, using wireless communication for health monitoring, fitness tracking, etc., can be part of Body Area Networks[7] | Provides health monitoring, fitness tracking, and real-time data analytics | Empowers individuals to manage their health proactively |
Implant | Usually, a medical device implanted in the body uses wireless communication to transmit data to external receivers. This may be part of Body Area Network (BAN) | Enables advanced medical monitoring and treatment | Enhances patient care through continuous monitoring |
Table 2-2: Hybrid Wireless Communications
Technology | Description | Impact | Human-Centricity |
RFID (Radio-Frequency Identification) | It uses electromagnetic fields to identify and track tags attached to objects automatically. | Streamlines logistics, inventory management, and security systems | Reduces human error and increases efficiency |
NFC (Near-Field Communications) | A set of communication protocols for communication between two electronic devices over a few (typically 4) cm or less | Facilitates secure contactless payments and data exchange | Simplifies daily transactions and improves security |
Table 2-3: Short Distance Communications
Technology | Description | Impact | Human-Centricity |
Zigbee | A specification for a suite of high-level communication protocols using low-power digital radios | Facilitates home automation and industrial control | Simplifies and automates various processes |
Z-Wave | A wireless communication protocol used primarily for home automation | Enhances home /industrial security and energy management | Creates smarter living environments |
Wi-Fi | Wireless networking technology for local area networks mainly uses IEEE 802.11 | Provides high-speed internet connectivity within local areas | Enables seamless access to the Internet and network resources |
Wireless Mesh | Network topology where nodes connect directly and dynamically | Ensures resilient and extensive local network coverage | Provides reliable connectivity in urban areas and large venues |
Table 2-4: Regional /Coverage Wireless Communications
Technology | Description | Impact | Human-Centricity |
WiMAX | High-speed broadband access over long distances | Suitable for areas where wired infrastructure is impractical | Provides internet access in remote or underserved regions |
Low Data Rates | See Table 2-5 | ||
Drones /UAV | Use wireless communications for remote control and data transmission | Facilitates agricultural monitoring, surveillance, and delivery services | Increases efficiency and accessibility in diverse fields |
Cellular | Cellular networks built upon wireless communications are overseen today by the 3GPP consortium[8]. The 3GPP consortium plays a crucial role by providing specifications as the foundation for developing and expanding these networks. These networks have evolved significantly since introducing 2G (GSM) technology.
The perspective provided in the diagram is the evolution from 2G to 5G(Frauendorf & Almeida de Souza, 2023). Cellular communications are today ingrained in almost every aspect of our lives, having a significant impact on our livelihood |
Table 2-5: Low Data Rates, Long-Range Wireless Communications
Technology | Description | Impact | Human-Centricity |
Sigfox | A global IoT network operator providing low-power, wide-area network (LPWAN) services | Enables reach to underground objects over large areas | Enhances sustainability for low-power objects |
NB-IoT (Narrowband Internet of Things) | a low-power wide-area (LPWA) technology developed to allow for a wide range of new IoT devices and services, mainly focussed on indoor coverage | Potentially enabling a wide range of applications that improve quality of life, enhance safety and security, increase efficiency, and contribute to sustainability | Enhances efficiency and sustainability in various indoor systems /environments |
LoRa (Long Range) | A spread spectrum modulation technique derived from chirp spread spectrum (CSS) technology. It has a typical range between 13 – 15 KM. | Supports IoT applications in agriculture, smart cities, and environmental monitoring | Enhances efficiency and sustainability in various sectors |
Table 2-6: Long Distance Wireless Communications
Technology | Description | Impact | Human-Centricity |
Satellites | GEO (Geostationary Earth Orbit): Satellites that orbit the Earth at the same rate as the planet rotates, staying in a fixed position relative to the surface.
MEO (Medium Earth Orbit): Satellites that orbit at altitudes between low and geostationary orbits. LEO (Low Earth Orbit): Satellites that orbit relatively close to Earth. HEO (Highly Elliptical Orbit): Satellites in an elliptical orbit with varying altitudes. Polar Orbit: Satellites that pass over the Earth’s poles, providing global coverage. |
Ensures global connectivity and positioning services | Provides communication and navigation support globally |
Deep Space | Communications for space exploration and interplanetary missions | Supports scientific research and space missions | Advances knowledge and inspires technological innovations |
Wireless communication systems, whether cellular, satellite-based, or other types, typically consist of several core components that enable the transmission and reception of signals wirelessly. They usually follow a hierarchical architecture that includes access networks, backhaul, and core networks, as described briefly in Table 2-7 and illustrated in Figure 2-2.
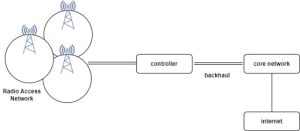
Table 2-7: Wireless Communications Hierarchical Architecture
Hierarchy | Wireless /Wi-Fi | Cellular | Satellite |
Access Network | Access points provide Wi-Fi connectivity to wireless devices (such as laptops, smartphones, and tablets) within a specific area, often referred to as a hotspot or coverage area. Each access point acts as an interface between wireless devices and the wired network infrastructure. | Access networks in cellular systems consist of base stations (cell towers) that communicate directly with mobile devices (e.g., smartphones and tablets). Each base station serves a specific geographic area known as a cell. Users connect to the cellular network via these base stations. | In satellite communication, access networks include ground terminals (earth stations) communicating with satellites in orbit. These ground terminals establish the link between the user equipment (satellite phones, terminals, antennas) and the satellite network. |
Backhaul | Backhaul refers to the connection between access points and the wired network infrastructure that provides internet connectivity in Wi-Fi networks. This connection can be wired (e.g., Ethernet) or wireless (e.g., point-to-point wireless links, microwave links). | In cellular systems, backhaul connections typically use fiber optic cables, microwave links, or other high-capacity connections to transport data from base stations to mobile switching centers (MSCs) or directly to the core network. | For satellite communication, backhaul connections involve transmitting data from ground terminals to satellite gateways or teleport facilities on Earth, which then connect to the core satellite network. |
Core Networks | Wi-Fi networks typically do not have a distinct core network in the same sense as cellular networks. Instead, the backhaul connections from access points usually terminate at a network router or gateway that connects to the broader Internet or a corporate network. | The core network is the central hub of the communication system where traffic from various access points and backhaul networks is routed and managed. It provides essential functions such as routing, switching, authentication, billing, and interconnection with other networks (e.g., the public switched telephone network (PSTN) or the Internet). | The core network in satellite communication encompasses ground-based facilities known as satellite gateways or earth stations. These are vital hubs connecting satellite systems to terrestrial networks, managing traffic routing and network operations, and ensuring seamless connectivity across global satellite constellations. Core network functions include monitoring satellite health, optimizing resource allocation, and facilitating secure data transmission between satellite terminals and terrestrial endpoints, supporting applications like broadband internet access, remote sensing, and global communication services. |
Internet | This represents the connection from the wireless core network to the broader Internet, allowing mobile devices to access Internet services and external networks. |
This hierarchical structure ensures that the network is scalable, manageable, and capable of providing reliable services to mobile users. Each layer has specific roles and responsibilities, contributing to the overall functionality and performance of the wireless network system.
Understanding Cellular Connectivity
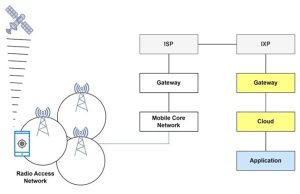
We discussed physical and digital spaces in the previous section. One of the areas where these two spaces intersect is the idea of locating persons/people, resources, and assets in any geographical area. To simplify, we will call this locating an entity. We will use this as an example to highlight the impacts on humanity and human-centricity. We need to understand how the system connects to the Internet and processes data /information to do this.
The cellular system connects to the Internet through network components and protocols designed to facilitate data transmission between mobile devices and the broader Internet infrastructure(see, for example, (Penttinen, 2015)). Signal /data at the application on the device or the server (for example, hosted on the cloud) travels through multiple components, as illustrated in Figure 2-3.
Mobile Stations (MS)
Users use these devices, such as smartphones and tablets or IoT devices that communicate wirelessly with the network. These devices may need Bluetooth capability or an embedded GPS (Global Positioning System) sensor chipset to process precise locations. GPS is a satellite-based navigation system that provides location and time information anywhere on or near the Earth with an unobstructed line of sight to four or more GPS satellites. In Figures 2-3, the phone communicates with the satellites to determine the precise positioning (outputs of geographic location coordinates) for the application running on the device.
Radio Access Network (RAN)
Mobile devices communicate wirelessly with the RAN, which includes base stations (like gNBs in 5G or eNodeBs in 4G LTE) and antennas. These components manage the radio interface and connect mobile devices and the core network. Once data reaches the RAN, it is forwarded to the core network.
Core Network (CN)
The elements in the core network manage mobility, authentication, session management, and data routing for mobile devices within the cellular network. In 4G and earlier generations, this includes components like the Mobile Switching Center (MSC), Serving GPRS Support Node (SGSN) /Serving Gateway (SGW), and Packet Data Network Gateway (PGW). In 5G, the core network may include elements such as the Access and Mobility Management Function (AMF), Session Management Function (SMF), and others.
Gateway to External Networks
Some gateways connect to external networks within the core network, such as the Internet. These gateways manage the data exchange between the cellular and external IP networks, including other mobile networks, fixed-line networks, and cloud services. The gateways are crucial connection points between network components, ensuring smooth data transfer and network interoperability.
Table 2-8: Cellular Network Architecture Evolution
Generation | Core Network (CN) | Internet Connectivity |
2G /GSM | In 2G networks, the core network primarily consists of the Mobile Switching Center (MSC) and the Home Location Register (HLR). The MSC manages voice calls and essential signaling between mobile devices and other networks (like the Public Switched Telephone Network, PSTN)—the HLR stores subscriber information, including service profiles and current location information. | 2G networks were primarily designed for voice communication and SMS. Internet connectivity was limited and typically provided through circuit-switched data services like CSD (Circuit Switched Data). Dial dial-up connections allow Users to access essential internet services via GPRS (General Packet Radio Service). Still, data speeds were prolonged, and the experience was not optimized for internet browsing or multimedia content. |
3G /UMTS | 3G networks introduced a packet-switched core network architecture. The core components include the MSC (which now handles both circuit-switched and packet-switched services), the Serving GPRS Support Node (SGSN), and the Gateway GPRS Support Node (GGSN). The SGSN manages mobility and session management for packet-switched data services. At the same time, the GGSN acts as the gateway between the mobile network and external IP networks (such as the Internet). | With 3G, mobile data services improved significantly. Users could access the Internet directly through the GGSN, which provides IP connectivity to external networks. This allowed for faster data speeds, always-on connectivity, and better support for internet-based applications such as web browsing, email, and early forms of mobile apps. |
4G /LTE | 4G /LTE networks have evolved packet core (EPC) architecture. Key components include the Evolved Packet Core (EPC), which includes the Serving Gateway (SGW), the Packet Data Network Gateway (PGW), and the Mobility Management Entity (MME). The SGW manages user data sessions within the LTE network, while the PGW acts as the interface between the LTE network and external IP networks (including the Internet). The MME handles signaling and manages mobility between LTE and other networks. | 4G /LTE provides high-speed data connectivity optimized for internet services. The PGW is crucial in connecting users to the Internet, providing IP address assignment, data routing, and quality of service (QoS) management. This architecture supports bandwidth-intensive applications such as HD video streaming, online gaming, and real-time communication services like VoIP. |
5G | 5G networks use a Service-Based Architecture (SBA) for the core network. Core components include the Access and Mobility Management Function (AMF), the Session Management Function (SMF), the User Plane Function (UPF), and others. The AMF manages access, mobility, and security functions. The SMF handles session management and policy enforcement, while the UPF manages user data forwarding. | 5G networks are designed to provide ultra-high-speed data connectivity, ultra-low latency, and support for massive IoT deployments. The UPF is the anchor point for user data sessions and connects external networks, including the Internet. 5G enables advanced use cases such as augmented reality (AR), virtual reality (VR), autonomous vehicles, and industrial IoT applications that require high reliability and low latency. |
Internet Service Providers (ISPs)
Data from the cellular network is routed through ISPs[9], which provide access to the internet backbone(Heckmann, 2006). ISPs manage the routing of data packets across their networks and ensure that data reaches its intended destination on the Internet.
Internet Exchange Points (IXP)
IXP[10],[11] are physical locations where multiple ISPs and networks interconnect to exchange traffic. They improve network efficiency, reduce latency, and provide direct peering between different networks, including those connecting from RANs to cloud services.
Additional Concerns
IP Address Assignment
Each mobile device is assigned an IP address[12], either dynamically or statically, which allows it to communicate over the Internet. IP address management and allocation are handled by network components such as the Dynamic Host Configuration Protocol (DHCP) server within the core network. Considering the exhaustion of IPv4 addresses[13], IPv6 deployments are on the rise for mobile Internet.
Data Transmission
Once connected to the Internet, mobile devices can send and receive data packets using standard protocols such as TCP/IP[14] (Transmission Control Protocol/Internet Protocol). These protocols ensure reliable communication between devices and facilitate web browsing, email, streaming media, and online gaming services.
Security and Authentication
Various security measures are implemented throughout this process to protect data transmission and user privacy. These include encryption, authentication mechanisms, and secure tunneling protocols like IPsec (Internet Protocol Security) and SSL/TLS (Secure Sockets Layer/Transport Layer Security).
Cellular Connectivity and Flow
From Mobile Device to RAN: The mobile device connects to the nearest base station in the radio access network.
From RAN to Mobile Core Network: The data from the mobile device is transmitted via the base stations to the mobile core network.
From Mobile Core Network to ISP: The mobile core network routes the data to the ISP through a gateway.
From ISP to IXP: The ISP connects to the IXP to exchange data with other networks.
From IXP to Cloud and Applications: The data can then be routed to cloud services or specific applications via additional gateways at the IXP.
Holistic View of Network Architecture
Telecommunication networks refer to the infrastructure and systems that allow for the transmission of voice, data, and video over distances. These networks can be interconnected in various ways to enable seamless communication globally. The Internet has significantly enhanced the convergence of these networks. This convergence has produced more efficient, flexible, and interconnected communication infrastructures worldwide.
One such view is presented in Figure 2-4. The various entities providing communication services and telecommunication technologies are presented in this illustration. The diagram was built to showcase the complexity and how growth is fostered using IXP, enabling faster and more reliable data transmissions. The illustration showcases comprehensive connectivity, allowing diverse networks and service providers to interoperate and facilitate efficient and reliable internet services.
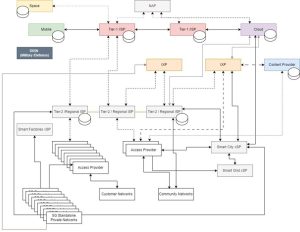
Here is a concise description of various elements and their relationships:
Space and Mobile Networks
Space: This likely represents satellite communication networks that can connect to terrestrial networks.
Mobile: Represents cellular networks, connecting mobile devices to the more extensive internet infrastructure.
DISN (Defense Information Systems Network)
Military/Defense: A specialized network for military and defense communications.
NAP (Network Access Point)
Central points where different networks connect and exchange traffic.
Tier-1 ISPs
These are large internet service providers that have a global presence and connect at NAPs and IXPs. They form the backbone of the Internet.
IXPs (Internet Exchange Points)
Points where multiple ISPs connect to exchange traffic, ensuring efficient data routing and reducing latency.
Tier-2/Regional ISPs
Smaller ISPs that primarily serve regional or national areas. They connect to Tier-1 ISPs and regional ISPs to access the global Internet.
Access Providers
These are local ISPs or other providers that offer internet access to end-users, such as homes and businesses.
Customer Networks
Networks owned by individual customers, such as businesses or large organizations. They connect to access providers for internet services.
Community Networks
Networks set up by local communities often provide internet access in areas where commercial providers are absent or insufficient.
5G Standalone Private Networks
These private networks use 5G technology for specific enterprises or industrial purposes.
Smart Factories xSP
Service providers cater specifically to intelligent factories, offering connectivity solutions tailored for industrial automation and IoT (Internet of Things) devices.
Smart City xSP
Service providers that focus on smart city infrastructure, providing connectivity for various urban applications like traffic management, public safety, and utility services.
Smart Grid xSP
Service providers that support innovative grid applications, facilitating advanced electricity distribution and management systems.
Cloud and Content Providers
Cloud: Represents cloud service providers offering computing resources and services over the Internet.
Content Provider: Entities that create and distribute content, such as streaming services, websites, and media companies.
Key Points of Connectivity
The diagram shows interconnections between these components, illustrating how data travels through various network layers.
Tier-1 ISPs connect directly to IXPs, which connect to Tier-2/Regional ISPs.
Content providers and cloud services are connected through IXPs, highlighting the importance of these points in efficiently distributing content.
Access Providers connect end-users to the more extensive Internet through Tier-2/Regional ISPs.
Specialized networks for intelligent factories, smart cities, and smart grids are integrated into the broader network, ensuring they can access and interact with other components as needed.
Wrap-up
This chapter has provided a cornucopia of mobile technologies that are ubiquitous in our lives. In the theater of technology, we can expect the most growth and interconnectivity to be witnessed, the highest number of new investments, and perhaps the most benefit to humanity.
References
Alessandretti, L., Aslak, U., & Lehmann, S. (2020). The scales of human mobility. Nature, 587(7834), 402–407. https://doi.org/10.1038/s41586-020-2909-1
Barbosa, H., Barthelemy, M., Ghoshal, G., James, C. R., Lenormand, M., Louail, T., Menezes, R., Ramasco, J. J., Simini, F., & Tomasini, M. (2018). Human mobility: Models and applications. Physics Reports, 734, 1–74. https://doi.org/10.1016/j.physrep.2018.01.001
Bluetooth Technology Website | The official website of Bluetooth technology. (n.d.). Bluetooth® Technology Website. Retrieved June 28, 2024, from https://www.bluetooth.com/
Frauendorf, J. L., & Almeida de Souza, É. (2023). The Evolution of Cellular Technologies. In J. L. Frauendorf & É. Almeida de Souza (Eds.), The Architectural and Technological Revolution of 5G (pp. 15–33). Springer International Publishing. https://doi.org/10.1007/978-3-031-10650-7_3
Griffiths, D. J. (2017). Introduction to Electrodynamics. Cambridge University Press. https://doi.org/10.1017/9781108333511
Heckmann, O. (2006). The Competitive Internet Service Provider: Network Architecture, Interconnection, Traffic Engineering and Network Design. In The Competitive Internet Service Provider. John Wiley & Sons, Ltd. https://doi.org/10.1002/9780470017906.fmatter
IEEE802. (n.d.). Retrieved June 28, 2024, from https://www.ieee802.org/
Kalmanek, C. R., Misra, S., & Yang, Y. (Eds.). (2010). Guide to Reliable Internet Services and Applications. Springer. https://doi.org/10.1007/978-1-84882-828-5
Miao, G., Zander, J., Sung, K. W., & Ben Slimane, S. (2016). Fundamentals of Mobile Data Networks. Cambridge University Press. https://doi.org/10.1017/CBO9781316534298
Penttinen, J. T. J. (2015). The Telecommunications Handbook. John Wiley & Sons, Ltd. https://doi.org/10.1002/9781118678916.fmatter
State of the Art of Small Spacecraft Technology. (n.d.). Retrieved June 28, 2024, from https://www.nasa.gov/smallsat-institute/sst-soa/soa-communications/
End Notes
[1] https://www.fcc.gov/engineering-technology/policy-and-rules-division/general/radio-spectrum-allocation
[5]https://www.itu.int/en/mediacentre/backgrounders/Pages/itu-r-managing-the-radio-frequency-spectrum-for-the-world.aspx
[6] For example, refer to this website for short-wave radio bands: http://www.short-wave.info/index.php
[9] An ISP is a company that provides internet access and connects users to the global network. This connectivity enables users and devices access to resources such as locations, web pages, and emails. Many telecommunication and internet standards organizations and heavily regulated industries support this complex business ecosystem. There are some excellent references to explore on ISP (Heckmann, 2006; Kalmanek et al., 2010)
[10] Internet Society, the early pioneers of the Internet transitioning from ARPANET and leading the way to make the Internet accessible to everyone in the world, has a short post on what an IXP is: https://www.internetsociety.org/resources/doc/2020/explainer-what-is-an-internet-exchange-point-ixp/
[11] APNIC has a good blog post on the history of IXP at https://blog.apnic.net/2019/07/19/shaping-the-internet-history-and-impact-of-ixp-growth/
[14] while SS7, signaling system 7, was predominant in early cellular systems for signaling, the adoption of TCP/IP began in the late 1990s and early 2000s with the advent of mobile data services like GPRS. Since then, TCP/IP has become the standard for handling data communication across cellular networks, complementing and sometimes replacing SS7 for various functionalities